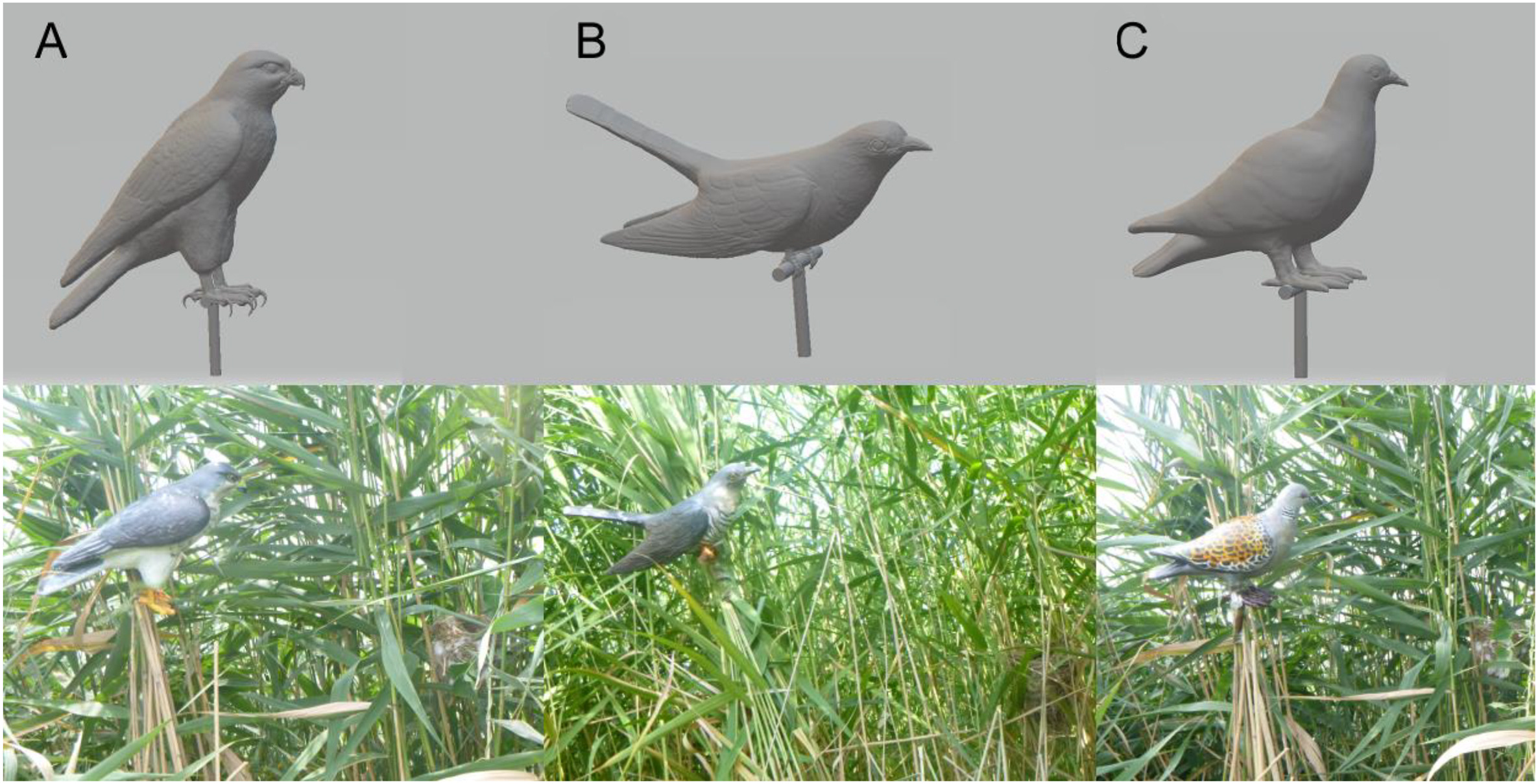
Citation: | Xiangyang Chen, Yan Cai, Jiaojiao Wang, Canchao Yang. 2022: Use of 3D-printed animal models as a standard method to test avian behavioral responses toward nest intruders in the studies of avian brood parasitism. Avian Research, 13(1): 100061. DOI: 10.1016/j.avrs.2022.100061 |
Living and/or non-living animal models are often used as stimuli to observe the behavioral responses of the target animals. In the past, parasites, predators, and harmless controls have been used to test host anti-parasitism defense behavior, and their taxidermy specimens have been widely used as a set of standard methods for the study of avian brood parasitism. In recent years, with the rapid development of 3D-printing technology, 3D-printed bird models are expected to be applied as a standard method in the study of avian brood parasitism. To evaluate the use of 3D-printed models, this study tests the reaction of Oriental Reed Warbler (Acrocephalus orientalis) towards predators, parasites, or controls, and compares the reaction among different nest intruders and between taxidermy specimens and 3D-printed animal models. It was found that the Oriental Reed Warbler responded most aggressively to the parasite, followed by predator, and finally the control; the results were consistent between the reaction to taxidermy specimens and 3D-printed animal models, indicating that 3D-printed models could serve as a substitute for taxidermy specimens. We propose a series of advantages of using 3D-printed models and suggest them to be a standard method for widespread use in future studies of avian brood parasitism.
Studies of animal behavior are conducted with the assistance of a variety of objects, including living animals (Ladu et al., 2015; Lawson et al., 2021) and non-living models comprising many different materials (Antunes and Biala, 2012; Lawson et al., 2021). These objects serve as stimuli in studies of the behavioral responses of animals within and across species, such as to conspecific intruders, potential mates, predators, and brood parasites. Non-living models, which may serve as replacements for systems involving living animals, are currently used frequently in animal behavior research, and they can be produced in a variety of ways. These models include the following: 1) taxidermy specimens from which the fur and/or feathers have been preserved, the body cavity stuffed with fillers such as cotton, and which have been preserved in antiseptic have been used by most studies, and include both stuffed models made by the researchers themselves and those borrowed from museums (Gill and Sealy, 1996; Schulze-Hagen et al., 2003; Welbergen and Davies, 2008; Henger and Hauber, 2014; Lawson et al., 2020); 2) sculptural models, which are produced by craftspeople from wood or plastic and then painted in the appropriate colors (Welbergen and Davies, 2012; Thorogood and Davies, 2016); 3) freeze-dried models, which are the direct sublimation of the solvent from the frozen animal's body at ultra-low temperatures, and are more durable than taxidermy specimens and maintain a relatively lifelike appearance (Bazin and Sealy, 1993; Maizato et al., 2013; Shoffner and Brittingham, 2013); and 4) three-dimensional (3D) printed models produced using 3D-printing technology, which have developed rapidly in recent years because of their repeatable, precise, and standardized production qualities (Conner et al., 2014; Behm et al., 2018). Inevitably, 3D-printed models also have certain limitations: they are static, and thus unable to simulate living organisms; only small objects can be printed; and their materials they are made of may contain toxic chemicals or be difficult to degrade. However, these deficiencies can be addressed by installing robots inside the models, assembling multiple printed parts into a larger model, and using recyclable materials (Walker and Humphries, 2019).
Although 3D-printing technology has been widely used in some aspects of behavioral studies, it is rarely used in avian brood parasitism (ABP), especially for studies of behavioral reaction to nest intruders (but see Chaumont et al., 2021), not to mention being recognized as a standard methodology in this research domain. However, using models as research tools is important in studies of ABP. Because the parasitism includes a series of steps from approaching a host nest (Feeney et al., 2012) to laying a parasite egg (Peer and Sealy, 1999; Soler, 2014) and manipulating the host parent (Soler et al., 1995, 1999; Swan et al., 2015), testing the host response against the parasite and its egg is a key to understand the coevolutionary interaction between the host and parasite. For example, to test the recognition on parasite egg by the host, model eggs that simulate the parasite eggs were widely used for artificial parasitism. The standard method for this experiment was to use hand-made model eggs made of polymer clay (Antonov et al., 2009; Yi et al., 2020; Yang et al., 2022a, b). Hitherto, only few studies have used 3D-printed model eggs for artificial parasitism (Igic et al., 2015; Dainson et al., 2017; Hays and Hauber, 2018; Abolins-Abols et al., 2019; Yang et al., 2019; Butler et al., 2020; Luro et al., 2020; Hauber et al., 2021). Furthermore, to test the host response toward parasite approaching its nest for parasitism, researchers generally used taxidermy specimen as a standard method (Gill and Sealy, 1996; Welbergen and Davies, 2008; Henger and Hauber, 2014; Lawson et al., 2020; Yang et al., 2022a, b), while using 3D-printed animal model was rare and its feasibility has not been evaluated. For example, Chaumont et al. (2021) used 3D-printed cuckoo models for ABP studies, but they did not make a comprehensive comparison and evaluation between 3D-printed models and taxidermy specimens. The purpose of this study is to provide an applied example of the use of 3D-printed models that simulate avian predators and brood parasites to test the behavioral reaction of a widespread bird species, the Oriental Reed Warbler (Acrocephalus orientalis), and also to evaluate the feasibility and advantages of using 3D-printed animal models for studies of animal behavior. We also provide some suggestions for future studies on ABP with 3D-printed animal models.
We performed this study in Yongnianwa National Wetland Park (36°40ʹ–36°41ʹ N, 114°41ʹ–114°45ʹ E) during the breeding season (May–July) of the Oriental Reed Warbler (hereafter the Reed Warbler) in 2021. The study site is a wetland habitat in a temperate semi-humid continental monsoon climate in Hebei Province in China, with the Common Reed (Phragmites australis) being the major herbaceous plant. The annual average temperature is 12.9 ℃, and the annual average precipitation totals 527.8 mm (Wang and Yang, 2020). The Reed Warbler belongs to the family Acrocephalidae, Passeriformes (Zheng, 2017), and is one of the main hosts of the Common Cuckoo (Cuculus canorus) in the study area; previous studies have found a parasitic rate of 14.8% in this study population (Ma et al., 2018; Wang et al., 2021).
We used three types of 3D-printed animal models, one each of the Sparrowhawk (Accipiter nisus), Common Cuckoo, and Oriental Turtle Dove (Streptopelia orientalis). These were designed using 3D Studio Max 2015 for Windows (Autodesk Inc., California, USA) and printed on a Digital Light Processing (DLP) 3D printer (StarRAY SP-DD230, Sprintray Inc., Guangdong, China). The models were then painted according to the natural phenotype of the animals (Fig. 1). These species are all sympatric with the Reed Warbler. The Sparrowhawk is a predator that preys on adult Reed Warblers (Wang et al., 2021) and the Common Cuckoo (hereafter the Cuckoo) is a brood parasite and nest predator that can threaten the nest, but not the adult Reed Warbler (Yang et al., 2017). The Oriental Turtle Dove (hereafter the Dove) is harmless to the Reed Warbler and here served as a control.
In the experiment, each Reed Warbler nest during the early incubation stage (the first three days of incubation) received the three animal models above as stimuli, with 1-h intervals between every two models. Each model was randomly selected and placed at a distance of 0.5 m in front of a nest, at the same height as the nest. We recorded the reaction from the Reed Warbler over the next 5 min with a digital video recorder (DMC-TS30, Panasonic Corporation, Xiamen, China), dividing actions into attacking (score = 4), mobbing (score = 3), alarm calling (score = 2), and no reaction (score = 1). We recorded the strongest level of reaction (a higher score refers to a stronger level) if a Reed Warbler presented more than one reaction during the experiment. A result of no reaction refers to the Reed Warbler ignoring the decoy. To evaluate the use of 3D-printed animal models, we conducted another experiment with the same procedure except the animal models were replaced with taxidermy specimens, these being a classical method for testing the behavioral reactions of animals. To avoid pseudoreplication, two replicates of 3D-printed animal models or taxidermy specimens were randomly selected for the experiment. The Reed Warbler is sexually monomorphic, so we did not distinguish different individuals by sex during experiments.
A cumulative link mixed model (CLMM) was used to analyze the reaction of Reed Warbler toward different types of stimuli. In this experiment the reaction (score 1–4) was the response; the trial (3D-printed animal model vs. taxidermy specimens), stimuli (Sparrowhawk, Cuckoo, or Dove), and replicate (two per model or specimen) were included as the fixed effects; and the clutch size, egg-laying date (of first egg), and nest identity were used as the random effects. The interactions between trial and stimuli and between trial and replicate were also tested. A post-hoc test using the Tukey method for P-value adjustment was used for pairwise comparison between different stimuli according to the trial.
The Dove is harmless to the Reed Warbler, so it was used here as a reference for reaction comparison. We predicted that the Dove would elicit the least intense reaction out of the three stimuli. The Cuckoo is a brood parasite and predator to the nest, and while it can have a highly negative impact on the reproductive success of the Reed Warbler, it is not dangerous to the adult birds themselves. The Sparrowhawk is a bird of prey, threatening adult Reed Warblers. Therefore, we predicted that the warblers would react more strongly to the Cuckoo than to the Sparrowhawk, because the former should trigger a mobbing and attacking reaction that is effective against the Cuckoo but dangerous when used on the Sparrowhawk. Furthermore, we predicted that the Reed Warbler would react consistently to the specimens and 3D-printed models if the latter was feasible as a substitution for the former.
The reaction scores for the Dove, Cuckoo, and Sparrowhawk taxidermy specimens were 1.467 ± 0.516, 3.333 ± 0.816, and 2.267 ± 0.799, respectively (n = 15 for all). For the 3D-printed animal models, these values were 1.467 ± 0.516, 3.400 ± 0.632, and 2.133 ± 0.640 (n = 15 for all). As we predicted, the reaction intensity followed a gradient from Dove (lowest) to Sparrowhawk (medium) to Cuckoo (highest). CLMM analyses showed that neither the trial (Z = 0.228, P = 0.819) nor the replicate (Z = 0.134, P = 0.893) had any significant differences. However, the Reed Warbler's reactions to stimuli did differ significantly between Dove and Cuckoo (Z = 5.495, P < 0.001, CLMM) and between Dove and Sparrowhawk (Z = 3.841, P < 0.001, CLMM). No statistically significant differences were observed in the interaction between trial and stimuli, nor between the trial and replicate (Table 1). Pairwise comparison indicated significant differences between the reactions of the Reed Warbler to the Cuckoo and to the Sparrowhawk for both specimens (t = 4.398, P < 0.001, Post-hoc test) and 3D-printed models (t = 5.222, P < 0.001, Post-hoc test; Table 2).
Fixed effect | Reference variable | Compared variable | Estimate | SE | Z | P |
Trial | Specimen | 3D model | 0.134 | 0.585 | 0.228 | 0.819 |
Stimuli | Dove | Cuckoo | 3.816 | 0.694 | 5.495 | < 0.001 |
Sparrowhawk | 1.876 | 0.539 | 3.841 | < 0.001 | ||
Replicate | Replicate 1 | Replicate 2 | 0.053 | 0.398 | 0.134 | 0.893 |
Trial × Stimuli | Specimen × Dove | 3D model × Cuckoo | 0.110 | 0.646 | 0.169 | 0.865 |
3D model × Sparrowhawk | −0.217 | 0.645 | −0.336 | 0.737 | ||
Trial × replicate | Specimen × Replicate 1 | Model × Replicate 2 | −0.301 | 0.574 | −0.524 | 0.600 |
Random effect: clutch size, egg-laying date, and nest identity. |
Empty Cell | Estimate | SE | df | t | P |
Taxidermy specimens | |||||
Cuckoo vs. Dove | 1.867 | 0.243 | 84 | 7.696 | < 0.001 |
Cuckoo vs. Sparrowhawk | 1.067 | 0.243 | 84 | 4.398 | < 0.001 |
Dove vs. Sparrowhawk | −0.800 | 0.243 | 84 | −3.298 | 0.004 |
3D-printed models | |||||
Cuckoo vs. Dove | 1.933 | 0.243 | 84 | 7.97 | < 0.001 |
Cuckoo vs. Sparrowhawk | 1.267 | 0.243 | 84 | 5.222 | < 0.001 |
Dove vs. Sparrowhawk | −0.667 | 0.243 | 84 | −2.748 | 0.019 |
Our results indicated that the Reed Warbler was reacting to the taxidermy specimens and 3D-printed animal models consistently. This study thus supports the conclusion that the use of 3D-printed animal models to substitute specimens is a feasible means of testing animal behavior. We suggest that the use of 3D-printed animal models should be extended as a standard method for studies of host nest defense in ABP because of their clear advantages: (1) High sustainability: compared to living animals or taxidermy specimens, 3D-printed models are more sustainable in utilization because they are beneficial for the biological conservation. The taxidermy specimens need strict storage conditions and are easily damaged (e.g., destroyed by the host) during experiments (Davies and Brooke, 1988; Welbergen and Davies, 2008, 2012), which undoubtedly leads to a need for constant supplement. Such a requirement for specimens would involve commercial interests, which may have a negative effect on the natural animal population, especially in developing and underdeveloped countries. (2) High comparability: using 3D-printed models would increase the comparability between different studies because their postures can be controlled and standardized, allowing for specific criteria to be shared between different studies. For example, researchers around the world can share their 3D model documents through scientific references. By contrast, both the posture and color may vary across different studies that use animal specimens or handcrafted models because they differ between manufacturers and crafters. It is impossible to make highly consistent specimens or handcrafted models, even from the same craftsperson. (3) High controllability: for the 3D-printed models, it is easy to manipulate one of the characteristics while controlling for others, which allows us to test the animal response to a specific trait or a combination of some traits optionally and independently. This idea has been proposed for the use of 3D-printed model eggs, which allows the researchers to change the characteristics of model eggs in one dimension while controlling for others (Yang et al., 2019). For the animal models, they have more characteristics and are more complicated, which makes such controllability more significant. For example, to test the size effect of nest intruders on host response, we can simply manipulate the size of the animal models while keeping all the other traits consistent. Yu et al. (2020) tested such size effects on a host, but the taxidermy specimens they used differed across many other traits because the specimens were different parasite species. It is not possible to change some characteristics of the taxidermy specimens while controlling for others, but this is easy for 3D-printed animal models.
In summary, this study provides supportive evidence that 3D-printed animal models can be used as a standard method for studies of ABP. The use of 3D-printed animal models brings a series of advantages, which are beneficial for biological conservation and make research studies more comparable and controllable. We believe this standard method allows the researchers to collect more objective and scientific data, which could reveal the visual signal cues of host recognition in more detail and thus help us better understand the coevolution between brood parasites and hosts in ABP studies.
CY designed the study, and YC and CY carried out laboratory and statistical analyses. XC and JW performed field experiments. XC and CY wrote the draft manuscript, and CY improved the manuscript. All authors read and approved the final manuscript.
The experiments comply with the current laws of China. Fieldwork was carried out under the permission from the Yongniwa National Natural Park.
The authors declare that they have no known competing financial interests or personal relationships that could have appeared to influence the work reported in this paper.
This study was supported by the Education Department of Hainan Province (HnjgY2022-12), Hainan Provincial Natural Science Foundation of China (320CXTD437 and 2019RC189), the National Natural Science Foundation of China (32260127 and 31672303) to CY, and the Hainan Provincial Innovative Research Program for Graduates (Qhyb2021-55) to XC.
Bazin, R.C., Sealy, S.G., 1993. Experiments on the responses of a rejector species to threats of predation and cowbird parasitism. Ethology 94, 326-338.
|
Conner, B.P., Manogharan, G.P., Martof, A.N., Rodomsky, L.M., Rodomsky, C.M., Jordan, D.C., et al., 2014. Making sense of 3-D printing: Creating a map of additive manufacturing products and services. Addit. Manuf. 1-4, 64-76.
|
Hays, I.R., Hauber, M.E., 2018. How the egg rolls: a morphological analysis of avian egg shape in the context of displacement dynamics. J. Exp. Biol. 221, b178988.
|
Lawson, S., Leuschner, N., Gill, B.J., Enos, J., Hauber, M.E., 2020. Loss of graded enemy recognition in a Whitehead population allopatric with brood-parasitic Long-tailed Cuckoos. Avocetta 44, 3-10.
|
Lawson, S.L., Enos, J.K., Antonson, N.D., Gill, S.A., Hauber, M.E., 2021. Do hosts of avian brood parasites discriminate parasitic vs. predatory threats a meta-analysis. Adv. Stud. Behav. 53, 63-95.
|
Luro, A.B., Fernández-Juricic, E., Baumhardt, P., Hauber, M.E., 2020. Visual acuity and egg spatial chromatic contrast predict egg rejection behavior of American robins. J. Exp. Biol. 223, b2296909.
|
Peer, B.D., Sealy, S.G., 1999. Laying time of the bronzed cowbird. Wilson Bull. 111, 137-139.
|
Schulze-Hagen, K., Steinheimer, F., Kinzelbach, R., Gasser, C., 2003. Avian taxidermy in Europe from the Middle Ages to the Renaissance. J. Ornithol. 144, 459-478.
|
Wang, J., Ma, L., Chen, X., Yang, C., 2021. Behavioral and acoustic responses of the oriental reed warbler (Acrocephalus orientalis), at egg and nestling stages, to the common cuckoo (Cuculus canorus). Front. Ecol. Evol. 9, 705748.
|
Welbergen, J.A., Davies, N.B., 2008. Reed warblers discriminate cuckoos from sparrowhawks with graded alarm signals that attract mates and neighbours. Anim. Behav. 76, 811-822.
|
Yang, C., Møller, A.P., Liang, W., 2022b. Light matters: nest illumination alters egg rejection behavior in a cavity-nesting bird. Avian Res. 13, 100016.
|
Yi, T., Sun, Y., Liang, W., 2020. Potential cuckoo hosts have similar egg rejection rates to parasitized host species. Avian Res. 11, 34.
|
Zheng, G., 2017. A Checklist on the Classification and Distribution of the Birds of China, 3rd Edn. Science Press, Beijing.
|
Fixed effect | Reference variable | Compared variable | Estimate | SE | Z | P |
Trial | Specimen | 3D model | 0.134 | 0.585 | 0.228 | 0.819 |
Stimuli | Dove | Cuckoo | 3.816 | 0.694 | 5.495 | < 0.001 |
Sparrowhawk | 1.876 | 0.539 | 3.841 | < 0.001 | ||
Replicate | Replicate 1 | Replicate 2 | 0.053 | 0.398 | 0.134 | 0.893 |
Trial × Stimuli | Specimen × Dove | 3D model × Cuckoo | 0.110 | 0.646 | 0.169 | 0.865 |
3D model × Sparrowhawk | −0.217 | 0.645 | −0.336 | 0.737 | ||
Trial × replicate | Specimen × Replicate 1 | Model × Replicate 2 | −0.301 | 0.574 | −0.524 | 0.600 |
Random effect: clutch size, egg-laying date, and nest identity. |
Empty Cell | Estimate | SE | df | t | P |
Taxidermy specimens | |||||
Cuckoo vs. Dove | 1.867 | 0.243 | 84 | 7.696 | < 0.001 |
Cuckoo vs. Sparrowhawk | 1.067 | 0.243 | 84 | 4.398 | < 0.001 |
Dove vs. Sparrowhawk | −0.800 | 0.243 | 84 | −3.298 | 0.004 |
3D-printed models | |||||
Cuckoo vs. Dove | 1.933 | 0.243 | 84 | 7.97 | < 0.001 |
Cuckoo vs. Sparrowhawk | 1.267 | 0.243 | 84 | 5.222 | < 0.001 |
Dove vs. Sparrowhawk | −0.667 | 0.243 | 84 | −2.748 | 0.019 |