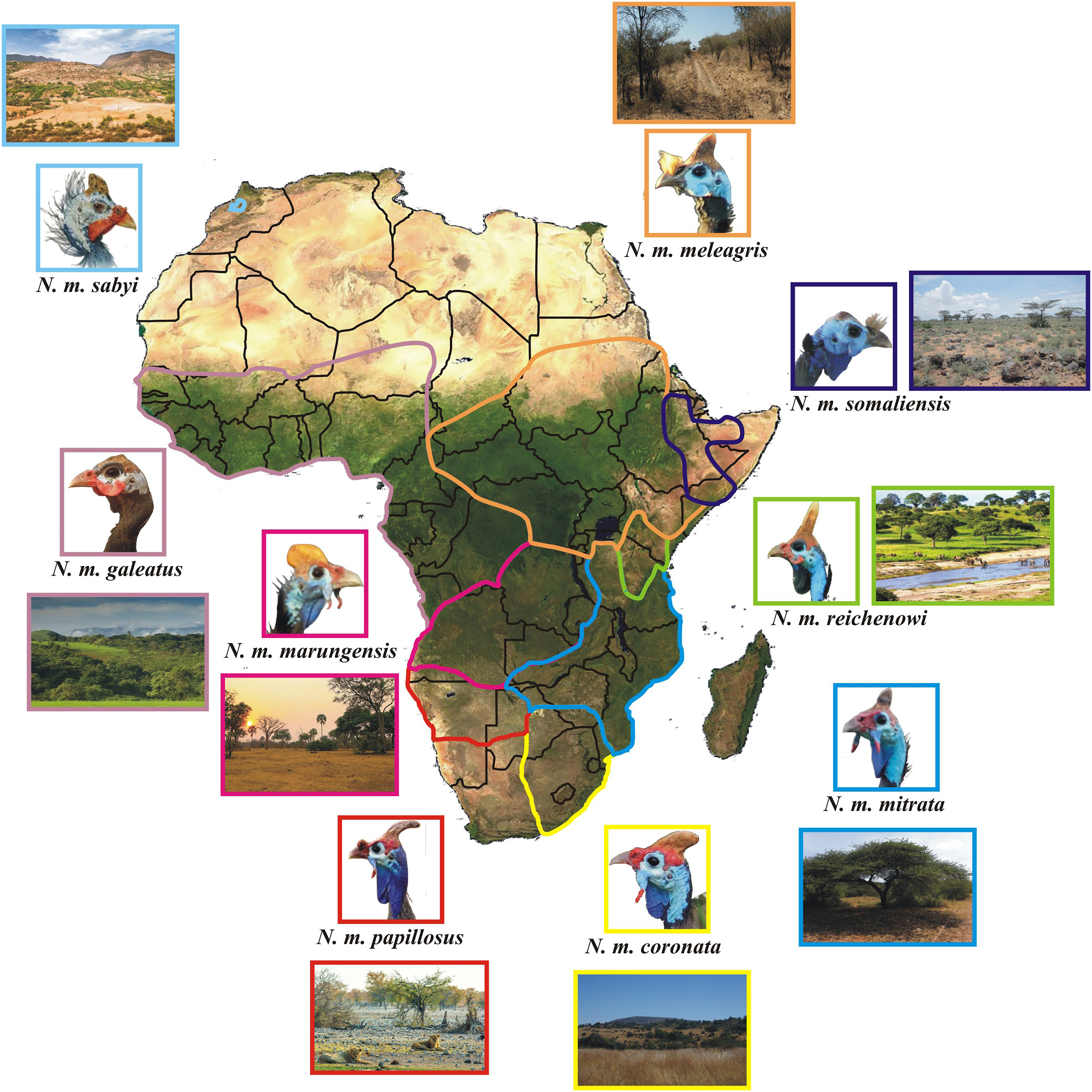
Citation: | Johann H. Van Niekerk, Rodrigo Megía-Palma, Giovanni Forcina. 2022: Thermoregulatory function and sexual dimorphism of the throat sack in Helmeted Guineafowl (Numida meleagris) across Africa. Avian Research, 13(1): 100047. DOI: 10.1016/j.avrs.2022.100047 |
The responses of ground-dwelling birds to heat and cold stress encompass a variety of behavioural, physiological and even morphological mechanisms. However, the role of glabrous skin in this respect has been marginally addressed so far. The Helmeted Guineafowl (Numida meleagris) is a landfowl distributed across Sub-Saharan Africa with eight traditionally recognised extant subspecies. Among the most prominent morphological traits underlying intraspecific variability are size and pigmentation of the bare throat skin (or sack), which might be related to the different habitats and environmental conditions across its wide range. In order to explore the Helmeted Guineafowl range-wide sack variation and pigmentation in relation to thermoregulation and sexual signalling, we collected morphometric and environmental information for N. m. coronata integrating field data with the inspection of photographic material encompassing seven subspecies and environmental information from their habitats. Field data evidenced that sack size was significantly correlated with ambient temperature, thus pointing to a likely involvement of the throat sack in thermoregulation. When the pictorial data from all subspecies were pooled, sack size correlated negatively with biomass, rainfall and humidity, while a positive correlation was found with annual solar irradiation. Sack size correlated positively with monthly temperature variation among the blue-throated subspecies from southern Africa as opposed to the black-throated subspecies ranging north to Zambia and Mozambique. Still, in this latter group the sack was often larger during winter months, possibly to maximise solar radiation absorbance. Noteworthy, sack size was related to sex dimorphism in two subspecies. Sack morphology and colour in the Helmeted Guineafowl likely modulate body temperature by evaporative cooling or heating upon needs, but in some subspecies it is also seemingly related to sexual signalling. Additional studies are needed to fully understand the multifunctionality of this important morphological feature in this species.
Birds have developed a suite of behavioural, physiological and even morphological responses to cope with heat stress (e.g., Carroll et al., 2015; Cook et al., 2020; Pessato et al., 2020; Fernandez et al., 2021; Kim et al., 2021; Playà-Montmany et al., 2021), which is particularly important for ground-dwelling species inhabiting extreme environments (Talbot et al., 2017). The absence or presence of tegumentary structures such as hairs or feathers and their features play an important thermoregulatory role in animals (Mota-Rojas et al., 2021). To date, however, the discussion about the purpose of bare facial and neck skin patches as well as of the pigmentation of these and other fleshy parts in birds has been centred on breeding phenology, social signalling and sexual selection (e.g., Van Niekerk, 1985, 2009; Kimball et al., 2008; Iverson and Karubian, 2017; Stuart-Fox et al., 2017), which is deemed to influence the evolution of morphological traits, particularly when sexual dimorphism is overt (Moore, 1990). Nevertheless, functional traits may evolve under different selection pressures, resulting into multifunctionality (Friedman et al., 2019).
Although the involvement of bare head and neck parts in sexual selection has been largely documented in birds, evidence points towards an important role of these structures also in thermoregulation through evaporative cooling and convection (Crowe and Withers, 1979; Stettenheim, 2000; Eduardo et al., 2010; Gerson et al., 2014; Galván et al., 2017). A pioneering study showed that the surface of the naked head and neck is adjusted to dissipate body heat in the American Black Vulture (Coragyps atratus) (Larochelle et al., 1982). Likewise, it is known that the Southern Ground Hornbill (Bucorvus leadbeatri) dissipates heat via the beak and unfeathered skin (Van Vuuren et al., 2020). Buchholz (1996) tested the heat-dissipation theory by insulating the bare heads and necks of Wild Turkeys (Meleagris gallopavo) with a hood, as if they were feathered, observing increased oxygen consumption, higher body temperature and decreased cooling capacity. However, little research has previously been undertaken to determine the role of bare facial colours in thermoregulation (Crowe, 1979; Walsberg, 1982; Kimball et al., 2008). Peculiar morphological features of head and neck may also have a thermoregulatory function. In a recent study, the long debated function of the peculiar helmet (or casque) of the Cassowary (Casuarius casuarius) has been indicated as primarily associated with thermoregulation, with this spongy bone acting as a thermal window (Eastick et al., 2019). It is possible that morphological traits originally developed to serve an antipredatory function evolved under different environmental pressures leading to their involvement also into homeostasis maintenance to cope with fluctuating ambient temperatures. This could be the case of the Helmeted Guineafowl (Numida meleagris), whose sponge-filled helmet was deemed to have originally evolved to protect eyes and skull of birds running away from predators through the scrub savannah (Crowe, 1978; Van Niekerk, unpublished). In some subspecies, it could be argued that this structure is also implied in thermoregulation, but supporting evidence has yet to be produced.
Interestingly, the Helmeted Guineafowl also displays bare throat skin, often hanging like a loose sack, that varies greatly in size and colour across its range. This raises the question about the adaptive meaning of this morphological trait and why different phenotypes evolved across morphological subspecies ranging in different habitats, which possibly points towards the effect of environmental factors. The implication of the throat sack in thermoregulation seems plausible since the neck hosts blood vessels involved in brain cooling (Crowe and Withers, 1979). By implanting thermocouples in the forebrain of Helmeted Guineafowl it was demonstrated that when the ambient temperature increases, their brain temperature also does, ranging between 36 and 42 ℃ in a healthy bird. Furthermore, heat radiation and convection experiments show that this species can lower its brain temperature through convective cooling of head and neck (Withers and Crowe, 1980) by stretching out the bare neck to dissipate heat (Crowe and Withers, 1979) while retracting it in the collar feathers when it is cold. Crowe (1979) studied morphological variation in Helmeted Guineafowl and showed how it relates to environmental variables, but no mention was made of the sack role in regulating the brain temperature. Nevertheless, in light of its polytypicity and distribution over a wide latitudinal range, the Helmeted Guineafowl is an excellent model to explore the alleged adaptive role of phenotypic variation (i.e., morphology and pigmentation) in the sack with a special focus on thermoregulation. A number of additional elements make the Helmeted Guineafowl a species of choice among other available ground-dwelling birds with bare throat skin to address this research topic. While the ground hornbills consist of just a couple of taxa, spurfowls (Pternistis spp.), which include as many as 24 species (Madge and McGowan, 2002), display bright yellow, orange or red skin patches which are not deemed to be related to heat absorption unlike darker colours (Stuart-Fox et al., 2017), being rather involved in sexual signalling (Van Niekerk, 1979, 2021). Moreover, spurfowl bare throats are inflatable (Van Niekerk, 2010) unlike those of Helmeted Guineafowl (Van Niekerk, 1983). Additional features that make this galliform a suitable model species are its sedentariety and high site-fidelity (with home ranges stretching over 1–2 km2) along the entire lifetime, which was inferred by means of mark-recapture experiments (Van Niekerk et al., 2009).
The Helmeted Guineafowl ranges across tropical and subtropical areas of Sub-Saharan Africa with eight extant morphological subspecies: N. m. coronata (southern Africa), N. m. papillosus (South West Africa), N. m. marungensis (Zambia, southern Democratic Republic of Congo, central and eastern Angola), N. m. mitrata (eastern and southeastern Africa), N. m. reichenowi (Tanzania and Kenya), N. m meleagris (Uganda, Sudan, Central African Republic, Chad, Ethiopia and Eritrea), N. m. somaliensis (Somalia) and N. m. galeatus (West-Central and Western Africa) (Fig. 1). One disjunct subspecies (N. m. sabyi) occurred in Morocco (Crowe, 1979) before it went extinct during the 1950s (Madge and McGowan, 2002; Birdfinding.info/helmeted-guineafowl/). For the sake of clarity, some authors assume the range of N. m. mitrata to include Limpopo Province of South Africa (Clancey, 1980; Chittenden et al., 2012), but we opted to rather follow Crowe (1978), who made a thorough investigation based on 1833 museum skins, and other authors (Madge and McGowan, 2002; Little, 2016).
The evolution process leading to present-day guineafowl across African biomes has been addressed in a seminal work based on multidisciplinary evidence pointing to the bare throat as a derived morphological feature (Crowe, 1978). In the early Pliocene, proto-Agelastes guineafowl with bare head and neck radiated into forests, which are now inhabited by species with fully feathered throats. Subsequently, the proto-Acryllium guineafowl moved into desert steppes, while proto-Guterra guineafowl moved into forest edge biotopes and proto-Numida guineafowl to savannahs. Each group speciated in this suite of different habitats under the selective pressures associated with different climatic conditions and landscape features, with several taxa developing specific neck and head structures presumably as adaptations to control brain temperature in arid and hot environments. For the proto-Numida group, this diversification process occurred in allopatry due to forest expansion isolating savannah patches. According to Crowe (1978), the typical morphological features of Numida, including the horny helmet, filoplumes (a likely protection against UV radiation) on the differently-coloured hindneck and the throat sack, are likely physioecological adaptations of former forest birds to cope with thermoregulation in open dry habitats but could also play a role in social interactions. Noteworthy, homeostasis maintenance and social signalling are crucial for the Helmeted Guineafowl, since this species forage over many hours (often up to 9 h per day) under direct sunlight. Since their food items are dry and often with poor nutritional value, guineafowl ingest about 25 g of biomass to obtain enough energy for roosting in trees at night, when temperature may drop to around ℃ in winter (Van Niekerk, unpublished). At the same time, the long time spent on the open ground for feeding exposes these birds to a high predation risk. For this reason, guineafowl form flocks of 15–40 birds that forage in closely knitted formations as an antipredator strategy (Crowe and Elbin, 1987), which shows why social signalling mediated by colourful bare skin patches is so important in this species.
In this study, we combined unpublished 40-year-old field data with material outsourced from a large online digital photographic archive and information from online climatic databases to explore the Helmeted Guineafowl range-wide sack variation and pigmentation in relation to thermoregulation and sexual signalling. For this purpose, we explored the involvement of environmental factors as possible drivers influencing the evolution of structural and colour variation in the Helmeted Guineafowl throat sack across a spatial gradient in Africa. Specifically, our purposes were to: (1) determine if sack size covaries with deep body temperature in the field; (2) correlate this information with sack area and colour across subspecies in response to different environmental variables; and (3) propose a scientifically plausible explanation of its role in thermoregulation.
From 1 March to 1 July 1982, 56 Helmeted Guineafowl (N. m. coronata) were trapped and marked with numbered metal rings in Krugersdorp Game Reserve (KGR: Gauteng, South Africa; 27°42ʹ41.14ʺ E, 26°5ʹ13.11ʺ S; Appendix Table S1; Van Niekerk, 1982). Birds were caught with a funnel walk-in trap (Van Niekerk, 2010), with the observers monitoring them 200 m away using binoculars to see when they entered it. Once in, the guineafowl kept feeding normally as if they were undisturbed. The trap was then approached as unobtrusively and quickly as possible and two people entered it, catching the birds to measure throat sack size and take body weight readings with a Persola spring balance scale (nearest gram). Guineafowl ring number, age (n = 56), mass (n = 28) and sex (n = 37) were recorded. We also measured the deep body temperature by means of a Hanna digital thermometer fitted with a thermocouple (needle probe) and placed about 5 cm deep into the vent. Measurements were recorded once the values stabilised on the digital screen of the thermometer, which took approximately 30 s (n = 15: young birds were excluded; see below) (Appendix Table S1). The surface temperature on the throat sack (Fig. 2A) was also recorded by enveloping it around the tip of the probe (about 10 mm long) with a self-made wooden forceps (to prevent heat convection). Multiple vent or skin temperatures per bird were not recorded to lessen stress (Nilsson et al., 2016). The surface of the throat sack was measured by unwrapping it gently as to assume its typical triangular shape against a white sizeable thin card box (shoebox). The sack outline was drawn with a pencil onto the paper, which allowed to determine its area (mm2) as that of a triangle calculated as half base multiplied by its height once linear measurements to the nearest mm had been taken with a vernier calliper. Only one side of the sack was measured and outlined (Fig. 2A). We also estimated its elasticity (i.e., sack variation across time) by sizing its surface up in adult birds both before (n = 18) and after (n = 13) handling (i.e., fitting individual rings, taking body temperature and sexing), which was an invariable source of stress. Importantly, the measurements pre- and post-handling were taken on different individuals and on different days. For the sake of clarity, the sack cannot be inflated or deflated: it is essentially a 1–2 mm thick flap which is relaxed when the weather is warm but tensed up around the neck when it is cold. At the same time, this structure is not deemed to be affected by gular fluttering, which is produced by pulsating the skin in the lower jaw with the mouth open (cf. Nassar et al., 2001). When Black-backed Jackals (Lupulella mesomelas) or predatory birds were observed near the trap, the guineafowl were not handled and measured as this would almost certainly further increased their stress levels, thus resulting in biased sack size measures. Field data collection took 6–10 min per bird (Appendix Table S1).
The photographs of 432 Helmeted Guineafowl (some of which captured in the same shot) stored in the Macaulay Library (Cornell Lab of Ornithology: www.macaulaylibrary.org) (Appendix Table S2) were included to show the variation in sack size along a spatial gradient (Fig. 1). The pictorial data of this repository are being used in other bird studies (e.g., Pyle, 2021), even though caution is needed, especially with digital images (Stevens et al., 2007 and references therein). We strived to select photographs shot as evenly as possible throughout the year in order to account for possible seasonal variation.
The inspection of this material also enabled to determine the sex of adult guineafowl (Appendix Table S3). Males have longer bony and uniformly apricot casques, slightly reddish at the base, which are often backward arching (Siegfried, 1966; Van Niekerk, unpublished), while in females casques — of the same colour — are shorter. Conversely, in first year birds of both sexes this morphological feature is markedly different, being fleshy and with reddish and blackish blotches (Van Niekerk, unpublished), with males starting to develop larger casques at the age of about 27 weeks (Siegfried, 1966). Males in breeding pairs often stand upright to spot predators (Van Niekerk, 1979; 2014; Prinsloo et al., 2005) and assume a low or high intensity hunchback posture when they threaten or chase each other (Crowe and Elbin, 1987; Van Niekerk, 1980), while females spend most of the time in a horizontal position.
No photographic material was found for the extinct N. m. sabyi from Morocco. The photos were selected as to have the lateral side of the head portrayed in the two-dimensional space (i.e., height and length) and minimise obliqueness (i.e., depth) around the edges, which could potentially bias measurements. Moreover, pictures of birds in alert posture, stampede mode or running were excluded since sack areas might have been affected by their stress condition (Van Niekerk, unpublished). Likewise, pictures of birds engaging in gular fluttering (for criteria cf. Nassar et al., 2001) were avoided because of the likely negligible yet possible effects on sack size. Photographs of N. m. coronata individuals west of its natural distribution in South Africa (Fig. 1) were avoided since these birds, introduced to the Western Cape over the past 150 years, are deemed to be admixed with domestic guineafowl (Clancey, 1980).
The sack invariably revealed itself as either a triangular or rectangular (most frequently observed in N. m. papillosus) shape (Fig. 2A), and a different approach was followed to obtain the surface depending on each case once linear measurements to the nearest mm had been taken with a vernier calliper. For triangles, the surface was calculated as the product of half base and height, while for rectangles as the product of their lengths and widths. In both cases, the side of the bare neck was calculated as a rectangle. For the sake of clarity, sack sizes were expressed as the percentage of the total bare skin visible in the picture (sack plus neck; only one side of the head). Measurements were taken from pictures of only seven extant subspecies since we could not find enough pertinent material dealing with N. m. somaliensis, for which, however, information on facial colours was obtained based on a couple of pictures (see acknowledgments).
In order to correlate monthly variation in ambient temperatures with monthly variation in sack size across subspecies, we gathered data from online climatic databases of meteorological stations from across their range (climate-data.com) and determined the mean monthly temperatures for each region. For the sake of clarity, the localities at issue included Skukuza and Bela Bela (South Africa) for N. m. coronata, Sossusvlei and Otjiwarongo (Namibia) for N. m. papilossus, Sofala (Mozambique) and Harare (Zimbabwe) for N. m. mitrata, Lusaka (Zambia) for N. m. marungensis, Nairobi and Tsavo West (Kenya) for N. m. reichenowi, Libreville (Gabon), Ziguinchor (Senegal) and Yaounde (Cameroon) for N. m. galeatus, and Addis Ababa (Ethiopia) for N. m. meleagris. Furthermore, we used D' Agostino test to ascertain that the frequency in the number of pictures selected per month and subspecies followed an unskewed distribution, which ensured an even representation of pictorial data across the year.
A wind factor was determined by multiplying the average monthly wind speed (km/h) by the average number of days per month during which wind was recorded (weather-and-climate.com/average). Humidity data were obtained from weather-and-climate.com/average and given as percentage. Annual direct normal irradiation (kWh/m2) (hereafter referred to as just annual solar irradiation) was sourced from World Bank (2019). Vegetation biomass values (Mg/ha) based on cover, canopy height and tree allometry information from Hanan et al. (2020) were implemented along with the precipitation values outsourced at https://commons.wikimedia.org/wiki/File:Africa_Precipitation_Map-sr.svg#file filelinks.
The sack size of males and female Helmeted Guineafowl trapped in KGR were compared using Kruskal-Wallis test (SciStatCalc.com). The same statistics was employed to assess possible variation in sack size as measured before and after bird handling. Spearman rank correlation (Social Science Statisticks.com) was used to explore the relation between sack size and deep body temperature of trapped birds.
We fitted the pictorial data on the sack size of five subspecies with a generalized linear model (GLM) with Gamma residual distribution and log as linking function. We included subspecies and sex of the birds as factor predictors in this model, as well as their interaction, to investigate whether significant differences in sack size between males and females could be generalized across seven N. meleagris subspecies (we had no data for N. m. somaliensis).
We also performed Mann-Whitney test comparisons of the sack size between subspecies pairs and calculated a correlation matrix with the ambient variables including annual solar irradiation (kWh/m2), biomass (Mg/ha), humidity (%) and wind factor (km/h). We confirmed the existence of a strong autocorrelation. Therefore, we summarised these variables in a single environmental factor (see results) by means of a principal component analysis (PCA). We employed a generalized mixed model with sack size as response, the environmental principal factor as fixed predictor and subspecies affiliation as random factor.
We also tested whether average sack size per subspecies covaried with monthly average minimum and maximum temperatures. Hence, we used a GLM where subspecies, maximum average temperature (ATmax), and minimum average temperature (ATmin) were set as predictors along with the interactions subspecies × ATmax and subspecies × ATmin to test if these relationships differed across taxa.
Sack size differed significantly between birds measured upon arrival at the trap and those handled later (i.e., 6–10 min). The average sack size before and after handling was 255.05 mm2 (n = 18, SD = 77.77, range = 93–400) and 314.23 mm2 (n = 13, SD = 77.12, range = 204–493), respectively; the difference was significant (n = 31, H = 3.92, P = 0.047). Six birds were captured twice and other two four times, each one on different days, revealing a variation in sack size (Appendix Fig. S1). We found a significantly negative correlation between sack size and deep body temperature (n = 15; rs = −0.067, P = 0.005; Fig. 2B, Appendix Table S1). In some adult birds, sex could not be determined, neither was it possible to determine the sack sizes or sex of birds aged 9–16 weeks (Appendix Table S1). Of the 56 Helmeted Guineafowl trapped in KGR, 15 were immature; of these, one — trapped in March — was a 9-week-old chick which, as such, possessed no throat sack, while head and facial parts were completely covered with natal down (cf. Siegfried, 1966), consistent with what is expected in the hatching season (January to March, namely the hot summer months in the southern hemisphere).
We also trapped seven chicks that were 14 to 16-week-old between March and June when, under cooler ambient conditions, the dark facial skins start appearing (cf. Siegfried, 1966), but no sack was present yet. However, this feature — first visible at the age of about six months — was present in some of the seven older juveniles trapped from June to July (the coldest months), and measured on average 186.9 mm2 (juveniles in Appendix Table S1: SD = 51.29, range 96–285.5). The sudden appearance of the throat sack in both sexes coincided with the loss of facial feathers in place of blue bare skin. No statistical difference in sack size emerged between males and females (n = 36, H = 0.01, P = 0.919; Appendix Fig. S2). Adult males weighting about 1150 g displayed the largest sacks, but their size receded as they got older and heavier (Fig. 2C).
The nine recognised subspecies fell in two main groups based on throat sack colour. The subspecies from the southern half of Africa, including N. m. coronata, N. m. papillosus, N. m. mitrata and N. m. marungensis, possess blue sacks. On the other hand, those from Central and East Africa, including N. m. reichenowi, N. m. galeatus and N. m. meleagris, possess black sacks. Finally, the disjunct N. m. sabyi in Morocco displayed mostly bluish-white facial parts (Madge and McGowan, 2002, Fig. 1). The representatives of the first group have long thin hanging wattles with red tips as opposed to their counterpart in which these are thick, rounded and either red or wholly blue (see Fig. 1). All the subspecies display blue bare facial colours around the eyes.
We found significant differences in sack size of N. m. meleagris when compared to N. m. papillosus and N. m. coronata, as well as in N. m. reichenowi when compared to N. m. papilossus and N. m. coronata (Fig. 3; Table 1). Furthermore, when inspecting the photographic material we could not see any throat sack (meaning that it was completely retracted around the neck) in some individuals of N. m. meleagris (29.09%), N. m. reichenowi (12.66%) and N. m. mitrata (1.2%), while it was always visible in N. m. papillosus and N. m. coronata.
χ2 | df | P-value | |
Sex | 10.7 | 1,256 | 0.001 |
Subspecies | 60.6 | 4,256 | < 0.001 |
Sex*Subspecies | 10.8 | 4,256 | 0.029 |
We determined the sex and the likely related sack size differences in 266 out of 432 photographed birds encompassing 5 subspecies (Appendix Table S2). We found significant sexual dimorphism (SSD) in sack size of N. m. meleagris and N. m. mitrata (Fig. 4; Table 2).
Pair | Mean Rank difference | Z | SE | Critical value | P-value |
N. m. coronata–N. m. meleagris | 179.488 | 5.973 | 30.049 | 91.292 | 2.328E-9 |
N. m. coronata–N. m. mitrata | 45.805 | 1.631 | 28.085 | 85.325 | 0.103 |
N. m. coronata–N. m. papillosus | 50.636 | 1.845 | 27.439 | 83.361 | 0.065 |
N. m. coronata–N. m. reichenowi | 143.226 | 5.346 | 26.789 | 81.387 | 8.971E-8 |
N. m. coronata–N. m. marungensis | 33.877 | 0.763 | 44.377 | 134.822 | 0.445 |
N. m. coronata–N. m. galeatus | 142.851 | 4.317 | 33.093 | 100.539 | 1.584E-5 |
N. m. mitrata–N. m. meleagris | -133.683 | 4.204 | 31.803 | 96.62 | 2.628E-5 |
N. m. papillosus–N. m. meleagris | -128.852 | 4.125 | 31.234 | 94.89 | 3.7E-5 |
N. m. meleagris–N. m. reichenowi | -36.262 | 1.183 | 30.664 | 93.161 | 0.237 |
N. m. marungensis–N. m. meleagris | -145.611 | 3.110 | 46.819 | 142.239 | 0.002 |
N. m. meleagris–N. m. galeatus | -36.637 | 1.009 | 36.302 | 110.287 | 0.313 |
N. m. mitrata–N. m. papillosus | 4.831 | 0.165 | 29.349 | 89.163 | 0.869 |
N. m. mitrata–N. m. reichenowi | 97.421 | 3.39 | 28.742 | 87.321 | 0.001 |
N. m. mitrata–N. m. marungensis | -11.928 | 0.262 | 45.583 | 138.484 | 0.794 |
N. m. mitrata–N. m. galeatus | 97.046 | 2.797 | 34.693 | 105.4 | 0.005 |
N. m. papillosus–N. m. reichenowi | 92.590 | 3.294 | 28.111 | 85.403 | 0.001 |
N. m. papillosus–N. m. marungensis | -16.759 | 0.371 | 45.188 | 137.283 | 0.711 |
N. m. papillosus–N. m. galeatus | 92.215 | 2.699 | 34.172 | 103.817 | 0.007 |
N. m. marungensis–N. m. reichenowi | -109.349 | 2.441 | 44.796 | 136.094 | 0.015 |
N. m. reichenowi–N. m. galeatus | -0.375 | 0.011 | 33.653 | 102.238 | 0.991 |
N. m. marungensis–N. m. galeatus | 108.974 | 2.232 | 48.828 | 148.343 | 0.026 |
SE: standard error; Z: Z-scores; PBonferroni: P-value after Bonferroni correction (0.002). |
The single principal factor derived from the environmental data (eigenvalue: 3.67) explained 73.36% of the initial variance in environmental variables. PC1 was positively correlated with humidity (0.86), rainfall (0.98) and biomass (0.80), whereas it was negatively correlated with annual solar irradiation (−0.92). Thus, when PC1 increased, humidity, rainfall and biomass did too as opposed to annual solar irradiation, which instead decreased. The general mixed model indicated a significant and negative relationship between sack size and the environment PC1 (β = −0.32 ± 0.04; Chi1,430 = 51.87; P < 0.001). Thus, birds with larger throat sacks are distributed in drier regions of Africa, with less vegetation and with higher annual solar irradiation (Fig. 5).
Concerning the monthly correlation between ATmin-ATmax and average sack size across subspecies, there was a significant effect of the subspecies by temperatures interaction (subspecies*ATmin: F4, 44 = 5.52, P = 0.001; subspecies*ATmax = F4, 44 = 2.64, P = 0.046). Hence, the three southernmost subspecies (i.e., N. m. mitrata, N. m. papillosus and N. m. coronata; see Fig. 1) showed a significantly positive correlation between sack size and both high and low ambient temperatures. However, the effects were stronger in relation with ATmin (Appendix Fig. S3, Table S3). On the other hand, no correlation was found for ATmin and ATmax in the northernmost black-throated subspecies.
The multifunctionality of bare facial and neck skin patches in birds has been addressed in several studies. However, while its role in sexual selection and social signalling has been widely explored, its involvement in thermoregulation is still scarcely investigated. In this context, wide-ranging polytypic species may represent insightful models to address important questions. In this study, we explored sack size variation in Helmeted Guineafowl subspecies encompassing different habitats and monthly varying conditions across Africa combining empirical data collected in the field with photographic material and environmental information stored in online repositories to elucidate the alleged adaptive significance of this trait. To the best of our knowledge, our work is among the first ones to rely on this novel approach which bears huge potential for future comparative studies. Noteworthy, the photographic repository allowed us to access a spatially representative and larger sample than the one we could have obtained based on museum skins, which, as noted by Crowe (1978), suffer from alterations of the original colours of naked body parts.
Our results are in line with the hypothesis that the heat dissipated through the sack of birds in the southernmost third of the African continent likely turns into lowering the temperatures of the supra-laryngeal, oesophageal, pharyngeal and vagus arteries situated just behind the sack around the throat, thus ultimately assisting cooling of brain and body (Crowe and Crowe, 1979). This function was specifically supported by the negative correlation of sack size with deep body temperature. At the same time, the blue-throated subspecies from this African region are known to withdraw the bare skin of the sack into the neck feathers to prevent heat loss from the brain when experiencing low ambient temperatures (Crowe and Withers, 1979). Concordantly, birds engage in sunning soon after coming from their roost trees during cold winter mornings with their bare necks and sacks exposed to the sunrays (Van Niekerk, unpublished), which suggests a possible role of these structures in transferring warm blood to the brain. This behaviour may also occur in the northern black-throated subspecies, in which a large sack is observed during cold months, with the dark pigments of the sack maximising the absorption of solar radiation (cf. Galván et al., 2018).
Immature individuals displayed no bare facial parts at hatching (Siegfried, 1966), with the natal down or feathers on their head and neck protecting them from solar radiation during summer months. Birds of this age are frequently observed to shelter under dense vegetation cover (Van Niekerk, 2010). The appearance of bare facial parts and sack in young birds during the height of winter invariably causes them to retract their neck into the collar feathers in the mornings and late afternoons to minimise the exposition of fleshy areas and retain body heat. On the other hand, adult birds are often observed standing with their necks outstretched and the sack rolled out when basking in the morning, supporting the idea that this behaviour maximises solar radiation absorbance to warm up the brain (Van Niekerk, unpublished). Young birds still have a seemingly limited ability to regulate their body temperature when ambient temperature fluctuates as observed in hatchlings of other species (Pereyra and Morton, 2001; Remeš and Martin, 2002; Nord and Giroud, 2020). Interestingly, the tendency of the throat sack to regress in older and heavier males can be imputed to a slower metabolism and, hence, an energy-preservation strategy similar to what was observed in the secondary sexual characters across other bird species (e.g., Møller and de Lope, 1999; Balbontín et al., 2011; Cornwallis et al., 2014) and in line with the reduction of thermogenic capacity in aging mammals (Florez-Duquet and Mcdonald, 1998; Grosiak et al., 2020). This phenomenon, presumably occurring also in older females, will require further elucidation.
Bare body parts may also work as social signals in birds by facilitating interactions between mates (Iverson and Karubian, 2017). Accordingly, we found significant SSD in the throat sack of N. m. meleagris and N. m. mitrata. However, this morphological trait was not sexually dimorphic in N. m. papillosus and N. m. coronata. In this respect, it should be noted that neither did we find a difference between the average sack size of N. m. coronata males and females trapped and measured in KGR, essentially showing consistency between fieldwork and photographic measurements. Admittedly, we could not test this SSD hypothesis in N. m. galeatus, N. m. marungensis, N. m. somaliensis and N. m. sabyi. The degree of sexual dimorphism in a species (i.e., phenotypic difference between sexes), is widely associated with the strength of sexual selection (Stuart-Fox and Ord, 2004; Fairbairn et al., 2008; Hoops et al., 2022). We have no evidence in this study to conclude that the latter varies across Helmeted Guineafowl subspecies, but it is arguable that different selective pressures may turn into lowering the degree of sexual dimorphism in some taxa.
The significant association among subspecies affiliation, sex and sack size supported the involvement of Helmeted Guineafowl throat sack in sexual signalling. This structure may have more than one function, assisting these birds in thermoregulation and mate choice (e.g., Iverson and Karubian, 2017; Tattersall et al., 2017; Van Jaarsveld et al., 2021). However, the lack of SSD in the other subspecies suggests that in some of the habitats occupied by N. meleagris across Africa the environmental pressures (e.g., climate) may exert a greater influence on sack size evolution than mate choice (Buchholz, 1996; cf. Galván et al., 2017).
The taxon-dependent multifunctionality of the sack is concordant with a multilocus galliform phylogeny postulating that bare facial parts play a role in heat loss but is also a product of sexual selection (Kimball et al., 2008). It appears that the SSD, occurring in some taxa only, developed as a secondary trait as opposed to the thermoregulatory function occurring in all subspecies. This interpretation is fully consistent with the scenario hypothesised by previous authors postulating that the plesiomorphic fleshy structures in galliforms were primarily involved in heat loss, possibly ending up with playing a role in sexual selection only at a later stage (Kimball et al., 2008, 2011).
Several lines of evidence suggest that the sack has a likely thermoregulatory function: (1) field data on N. m. coronata evidenced that its size correlates negatively with deep body temperature, which most likely suggests heat dissipation through a larger surface; (2) a positive correlation was found between sack size and monthly ambient temperature variation in the southern blue-throated subspecies, exposed to a sharper thermal variation across seasons; (3) even if such correlation was not found in the northern black-throated subspecies, the sack was larger during winter, which is likely to maximise heat absorbance; (4) the biggest sacks in the southern blue-throated subspecies were found in open and drier habitats, probably since larger skin surfaces assist evaporative cooling when less shade is available in the surrounding habitat.
Likewise, the pigmentation of throat sack as it manifests in all subspecies seems related to thermoregulation, with heat absorption facilitated by the dark colour of northern taxa. For instance, the interplay of black sack and less blue facial parts observed in N. m. galeatus aligns with Gloger's rule (Delhey, 2017), stating that birds inhabiting densely vegetated and humid habitats display dark colours (see also Crowe, 1979), which is also functional to camouflage. Black patches are thought to increase heat dissipation through evaporative cooling in humid tropical environments (Galván et al., 2018), but given their reduced size in N. m. galeatus, their role in regulating brain temperature must be limited and possibly constrained by other factors. Our contention is therefore that the lighter blue sacks of southern subspecies make them better adapted to sunny and windy open conditions by deflecting solar radiation while promoting evaporative cooling as compared to black throats, absorbing heat but constraining this process in their northern counterpart. The invariant occurrence of light blue colours around the eyes across all subspecies might be an adaptation to deflect the sunlight and prevent interference with vision (Jeren et al., 2019). Indeed, the limited vascularisation of this area would impair thermoregulation and, consequently, an increase of local temperature might be harmful.
The Helmeted Guineafowl dispersed throughout African open savannah during late Pliocene (Crowe, 1978). However, when forest expansion took place during early Pleistocene, some populations adapted to more densely vegetated areas around remaining forest patches (after a second spell of contraction) in the tropics, originating N. m. galeatus, while others settled in less vegetated regions, diverging into N. m. reichenowi and N. m. meleagris. Other subspecies remained in drier open habitats either in southern (N. m. coronata and N. m. papillosus) or northern (N. m. sabyi) Africa. In these environments, the bare facial skin and the throat sack with its thermoregulatory function can assist these birds in accessing and exploiting open areas away from vegetation patches. In other words, the Helmeted Guineafowl can feed in sunlight and remain cool headed. This also happens during winter, when the weather is dry, and food becomes scarce. In the southern third of Africa, temperature during winter afternoons can reach up to 25 ℃, so the sack would also serve to dissipate heat. Indeed, field observations in KGR showed that the sack is rolled out also during times of heat stress in winter or retracted during cold spells in summer after hailstorms (JHvN personal observations).
The Helmeted Guineafowl subspecies had hitherto never been classified into a southern blue-throated and a northern black-throated group (cf. Crowe, 1978), but it is strikingly evident how several homologous morphological characteristics and environmental correlations are associated with each one of these categories. The northern black-throated group is characterised by black facial parts, rounded wattles and smaller throat sacks. Their body temperature balance is seemingly less affected by ambient temperature, which varies more moderately in and around tropical Africa, than that of their counterpart. Conversely, the southern blue-throated subspecies from more open habitats have developed larger sacks to facilitate heat dissipation and the maintenance of homeostasis, with N. m. coronata and N. m. papillosus showing — unlike other subspecies — no evidence of involvement in sexual signalling.
The distinctiveness of northern and southern group suggests that they might have originated from separate ancestral lineages, and that their divergence was essentially influenced by vegetation biomass and other environmental variables among which ambient temperature played an important role (Crowe, 1979; Cooney et al., 2016). The discrete spatial patterns of phenotypic features such as wattle shape and colours could conceivably suggest that they diverged to minimise interbreeding between neighbouring subspecies, as in the case of N. m. galeatus and N. m. meleagris, but supporting genetic evidence has yet to be produced.
After the work by Crowe (1978), addressing the evolution of guineafowl as a whole, the only study attempting to make a reappraisal of the Helmeted Guineafowl intraspecific phylogeny was actually focused on elucidating the origin of domestic guineafowl (Shen et al., 2021). When using the Vulturine Guineafowl (Acryllium vulturinum) and the Crested Guineafowl (Guterra edouardi) as outgroups, the clade of wild eastern African Helmeted Guineafowl (Sudan and Kenya) diverged first, followed by wild conspecifics from Nigeria and, subsequently, the domestic guineafowl stock originated in West Africa between 5500 and 1300 years ago. However, an in-depth genomic study encompassing present-day N. meleagris subspecies as a whole is needed to make conclusive statements on the species diversification process and taxonomy. In this respect, the genome assembly that was recently produced for the domestic N. meleagris line (Vignal et al., 2019) may represent a useful resource, even though a wild individual would work better as reference.
Our results pointed towards a primary role of Helmeted Guineafowl sack morphology and pigmentation in thermoregulation, with homeostasis being putatively maintained by both evaporative cooling and deflection of solar radiation. On the other hand, observational data suggest that throat sack can assist the birds to gain heat during sunning in cold mornings. This process may be facilitated by the dense arteries of the sack and neck feeding blood to the brain and the rest of the body. We suggest that evaporative cooling is maximised by lighter pigments of the subspecies occurring in the southern third of Africa and inhabiting more open areas with higher wind factors. Conversely, the black-throated subspecies further north seems to be less impacted by ambient temperature, which, at those latitudes, is less prone to sharp fluctuations; this is the likely reason why a large sack is not required in these taxa. Our assertion is that the colour combinations, in conjunction with sack size, is functional to the modulation of heat absorption across the bare neck and facial parts, thus optimising the physiological functions of each subspecies in its own specific habitat.
The alleged thermoregulatory role, however, does not exclude an additional implication of sack size and colour with social signalling. Concordantly, sack size was sexually dimorphic in N. m. meleagris and N. m. mitrata, pointing to its implication in sexual selection (Mori et al., 2017). On the other hand, the absence of SSD of the sack sizes in the two most southerly subspecies, N. m. coronata and N. m. papillosus, could be likely ascribed to its overriding thermoregulatory role in regions where solar radiation and draught are more prominent - and homeostasis maintenance more energetically expensive - than elsewhere. Admittedly, the availability of real-time versus average monthly environmental data other than field information for the entire subspecies set would have provided a more convincing evidence supporting our results, which should be corroborated by further empirical research like the one conducted in KGR. Likewise, the comparison of local environmental climate data versus time-energy budget (i.e., individual metabolic rate) would further elucidate the function of the throat sack in guineafowl. Additional studies are needed to deepen our knowledge of the poorly explored field of thermoregulation in avian research.
JVN: conceptualisation, data curation, formal analysis, funding acquisition, investigation, writing – original draft, writing – review & editing. RMP: formal analysis, methodology, writing – review & editing. GF: formal analysis, methodology, writing – review & editing, funding acquisition, visualisation. All authors read and approved the final manuscript.
All operations were conducted with permits issued by the Department of Nature Conservation of the former Transvaal Provincial Authority. The Parks Board of Krugersdorp Municipality (currently Mogale City Parks and Recreation) provided permission to conduct research in KGR. JHvN was approved as a B-grade (no B 717) ringer by SAFRING, Cape Town University at the time of fieldwork.
The authors declare that they have no competing interests.
The authors are thankful to the following people for granting permission to use their guineafowl pictures or drawings (subspecies, Macaulay Library or other ID, if available, and locality are provided in parentheses along with the link in case of missing ID): Garry Douglas (N. m. marungensis, ML175816081, Zambia), Peter Hills (N. m. meleagris, BOA11804, Ethiopia), Nigel Voaden (N. m. reichenowi, ML46988951, Kenya), Gerard Vigo (N. m. galeatus, Gambia), Guenther Karmann (N. m. coronata, ML204783541, South Africa), Laurent Esselen (N. m. papillosus, ML240295901, Namibia), Montserrat Rubio Masero (N. m. sabyi, Morocco, https://www.pinterest.es/pin/854206254314512653/), Kenrith Carter (N. m. somaliensis, ML335872191, Somalia) and Wolfgang Dreier (N. m. somaliensis, https://www.biolib.cz/en/taxon/id444554/), Zak Pohlen (N. m. mitrata, ML276498351, Mozambique). The picture of N. meleagris (https://upload.wikimedia.org/wikipedia/commons/2/24/Numida_meleagris_-Ngorongoro_Conservation_Area%2C_Tanzania_-upper_body-8.jpg) used in Fig. 2 was freely usable under license CC BY 3.0. The authors are also grateful to the photographers who shared their habitat images: Sanjeev Arora (N. m. papillosus, https://www.blogger.com/profile/10896480884587733715), F. Gachathi (N. m. somaliensis, http://vecea.vegetationmap4africa.org/docs/S.html) and Vlada Trailin (N. m. meleagris, http://www.reptiles-of-ethiopia-and-eritrea.com/Echis-varlocV.jpg). The habitat picture of N. m. coronata was taken by JHvN and those of N. m. mitrata, N. m. galeatus and N. m. reichenowi were freely available at https://pixabay.com/photos/vegetal-cover-mozambique-5175462/, https://www.shutterstock.com/image-photo/landscape-bamenda-highlands-cameroon-africa-112305587 and https://www.shutterstock.com/image-photo/elephants-crossing-river-serengeti-national-park-379974949, respectively. Finally, the authors wish to thank Bertie van Niekerk and Frans Rossouw for their valuable assistance during fieldwork in KGR.
Supplementary data to this article can be found online at https://doi.org/10.1016/j.avrs.2022.100047.
Chittenden, H., Allan, D., Weiersbye, I., 2012. Roberts Geographic Variation of Southern African Birds. The John Voelcker Bird Book Fund, Cape Town.
|
Clancey, P.A., 1980. Checklist of Southern African Birds. The Southern African Ornithological Society, Pretoria.
|
Crowe, T.M., 1978. The evolution of guinea-fowl (Galliformes, Phasianidae, Numidinae) taxonomy, phylogeny, speciation and biogeography. Ann. S. Afr. Mus. 76, 43-136.
|
Crowe, T.M., Elbin, S.B., 1987. Social behaviour of Helmeted Guineafowl Numida meleagris. S. Afr. J. Wildl. Res. 1, 55-57.
|
Crowe, T.M., Withers, P.C., 1979. Brain temperature regulation in Helmeted Guineafowl. S. Afr. J. Sci. 75, 362-365.
|
Eduardo, J., Bicudo, P.W., Buttemer, W.A., Chappel, M.A., Pearson, J.T., Bech, C., 2010. Ecological and Environmental Physiology of Birds. Oxford University Press, New York.
|
Little, R., 2016. Terrestrial Gamebirds and Snipes of Africa. Jacana, Cape Town.
|
Madge, S., McGowan, P., 2002. Pheasants, Partridges and Grouse. Christopher Helm, London.
|
Mori, E., Mazza, G., Lovari, S., 2017. Sexual dimorphism. In: Vonk, J., Shakelford, T. (Eds. ), Encyclopedia of Animal Cognition and Behavior. Springer International Publishing, Switzerland, pp. 1-7.
|
Prinsloo, H.C., Harley, V., Reilly, B.K., Crowe, T.M., 2005. Sex-related variation in morphology of Helmeted Guineafowl (Numida meleagris) from Riemland of the northeastern Free State, South Africa. S. Afr. J. Wildl. 35, 95-96.
|
Siegfried, W.R., 1966. Growth, plumage, development and moult in Crowned Guineafowl Numida meleagris coronata Gurney. Depart. Nat. Conserv. Invest. Rep. 9, 1-52.
|
Van Niekerk, J.H., 1979. Social and breeding behaviour of the Crowned Guineafowl in the Krugersdorp Game Reserve. Ostrich 50, 188-189.
|
Van Niekerk, J.H., 1980. Some socio-biological features of Crowned Guineafowl in the Krugersdorp Game Reserve. Bokmakierie 32, 102-108.
|
Van Niekerk, J.H., 1982. Stalking and enticing guineafowl in a game reserve. Safring 11, 57-61.
|
Van Niekerk, J.H., 1983. Marking and observing Helmeted Guineafowl in the Krugersdorp Game Reserve. Safring 12, 48-53.
|
Van Niekerk, J.H., 1985. Observations on courtship in Swainson's Francolin. Bokmakierie 35, 90-92.
|
World Bank, 2019. Global Solar Atlas 2.0. Solargis.
|
χ2 | df | P-value | |
Sex | 10.7 | 1,256 | 0.001 |
Subspecies | 60.6 | 4,256 | < 0.001 |
Sex*Subspecies | 10.8 | 4,256 | 0.029 |
Pair | Mean Rank difference | Z | SE | Critical value | P-value |
N. m. coronata–N. m. meleagris | 179.488 | 5.973 | 30.049 | 91.292 | 2.328E-9 |
N. m. coronata–N. m. mitrata | 45.805 | 1.631 | 28.085 | 85.325 | 0.103 |
N. m. coronata–N. m. papillosus | 50.636 | 1.845 | 27.439 | 83.361 | 0.065 |
N. m. coronata–N. m. reichenowi | 143.226 | 5.346 | 26.789 | 81.387 | 8.971E-8 |
N. m. coronata–N. m. marungensis | 33.877 | 0.763 | 44.377 | 134.822 | 0.445 |
N. m. coronata–N. m. galeatus | 142.851 | 4.317 | 33.093 | 100.539 | 1.584E-5 |
N. m. mitrata–N. m. meleagris | -133.683 | 4.204 | 31.803 | 96.62 | 2.628E-5 |
N. m. papillosus–N. m. meleagris | -128.852 | 4.125 | 31.234 | 94.89 | 3.7E-5 |
N. m. meleagris–N. m. reichenowi | -36.262 | 1.183 | 30.664 | 93.161 | 0.237 |
N. m. marungensis–N. m. meleagris | -145.611 | 3.110 | 46.819 | 142.239 | 0.002 |
N. m. meleagris–N. m. galeatus | -36.637 | 1.009 | 36.302 | 110.287 | 0.313 |
N. m. mitrata–N. m. papillosus | 4.831 | 0.165 | 29.349 | 89.163 | 0.869 |
N. m. mitrata–N. m. reichenowi | 97.421 | 3.39 | 28.742 | 87.321 | 0.001 |
N. m. mitrata–N. m. marungensis | -11.928 | 0.262 | 45.583 | 138.484 | 0.794 |
N. m. mitrata–N. m. galeatus | 97.046 | 2.797 | 34.693 | 105.4 | 0.005 |
N. m. papillosus–N. m. reichenowi | 92.590 | 3.294 | 28.111 | 85.403 | 0.001 |
N. m. papillosus–N. m. marungensis | -16.759 | 0.371 | 45.188 | 137.283 | 0.711 |
N. m. papillosus–N. m. galeatus | 92.215 | 2.699 | 34.172 | 103.817 | 0.007 |
N. m. marungensis–N. m. reichenowi | -109.349 | 2.441 | 44.796 | 136.094 | 0.015 |
N. m. reichenowi–N. m. galeatus | -0.375 | 0.011 | 33.653 | 102.238 | 0.991 |
N. m. marungensis–N. m. galeatus | 108.974 | 2.232 | 48.828 | 148.343 | 0.026 |
SE: standard error; Z: Z-scores; PBonferroni: P-value after Bonferroni correction (0.002). |