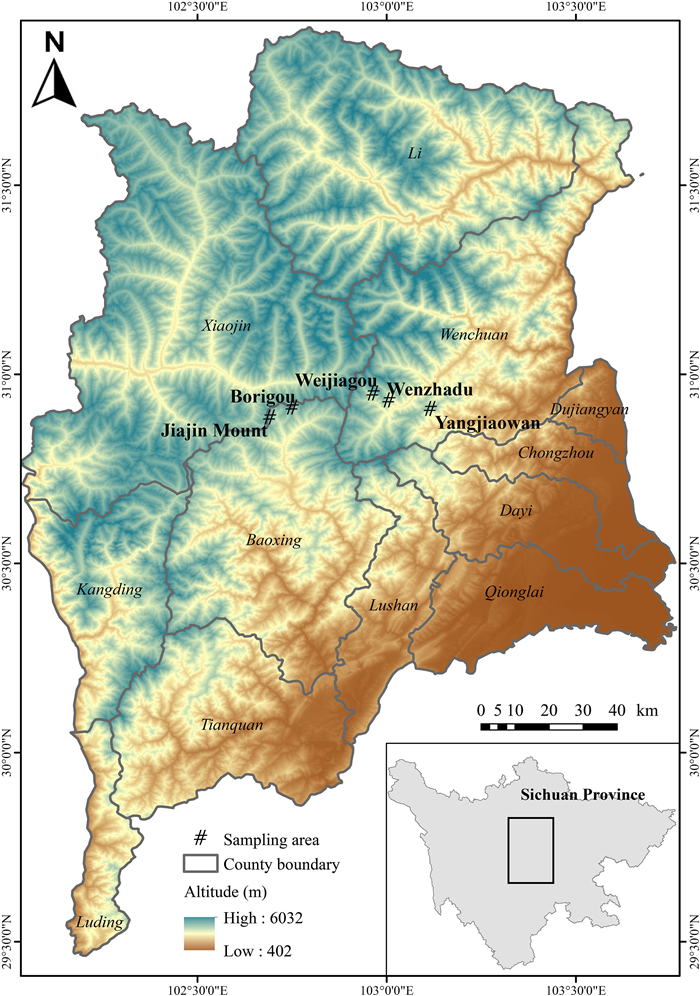
Citation: | Bin Wang, Yun Li, Guiquan Zhang, Jian Yang, Cao Deng, Haoyu Hu, Long Zhang, Xiaoqin Xu, Caiquan Zhou. 2022: Seasonal variations in the plant diet of the Chinese Monal revealed by fecal DNA metabarcoding analysis. Avian Research, 13(1): 100034. DOI: 10.1016/j.avrs.2022.100034 |
The Chinese Monal (Lophophorus lhuysii) is an alpine-obligate galliform species of global conservation priority. It has been listed as a first class protected wildlife species in China, requiring conservation actions during the 14th Five-Year Plan period. However, the diet composition of Chinese Monal and its seasonal variations have rarely been studied, constraining the effective conservation of the species. Here, we investigated the plant diet composition of the Chinese Monal and its seasonal variations using a DNA metabarcoding approach on fecal samples. We collected 190 fecal samples of the Chinese Monals from the central Qionglai Mountains located in China, and analyzed the plant diet of this species using a DNA metabarcoding approach. Taxonomic profiling of higher plants in the fecal samples was performed using the second internal transcribed spacer (ITS2) amplicon. Downstream analyses, including rarefaction curves, nonmetric multidimensional scaling (NMDS) and permutational multivariate analysis of variance (PERMANOVA), were used to explore the seasonal variations in diet composition. The Chinese Monal foraged a wide range of plant recipes composed of 35 families and 83 genera throughout the year, with Brassicaceae, Apiaceae, and Poaceae as the dominant families, and Cardamine as the dominant genus. The species consumed plants from 62 genera from 28 families during the breeding season (n = 81) and 66 genera from 31 families during the non-breeding season (n = 109). Further, the plant diet composition significantly varied between the breeding and non-breeding seasons, especially for the frequency of occurrence and relative read abundances at genus level. Our study analyzed the plant diet of the Chinese Monal at a high resolution for the first time, and the results revealed that the seasonal variations in its plant diet composition was adapted to plant phenology and foraging strategy. Fritillaria species, a previously confirmed important food resource for the Chinese Monal, were not detected in any fecal samples, potentially due to overharvesting of Fritillaria bulbs for Traditional Chinese Medicine. Therefore, we highly recommend further restriction of herb gathering in Chinese Monal habitats to facilitate the conservation of this endangered species. Altogether, our study enriches essential ecological information for the Chinese Monal and also provides insights into conservation management for this endangered species.
Food is the most fundamental resource for animal survival. Species evolve specific diets that are adapted to their nutrient requirements and environmental resources, and they adapt their diet in response to phenological changes, interspecific interactions, disturbances, and other external pressures (Gainsbury and Meiri, 2017; Gül and Griffen, 2020). In particular, resident species with low mobility are sensitive to local fluctuations of food resources, as they are not able to obtain foods over long distances, and food shortages play a critical role in regulating their populations (Kluen et al., 2017). Therefore, understanding the diet of an endangered species is essential for identifying resource requirements and the potential factors related to its population depression, and is thus important for conservation management (Ward et al., 2012; Shao et al., 2021).
The Chinese Monal (Lophophorus lhuysii) belongs to the family Phasianidae, order Galliformes. As an alpine-obligate species endemic to China, the Chinese Monal has a narrow distribution range that is restricted to the eastern edge of the Qinghai-Tibet Plateau (Xu et al., 2020) and they mainly inhabit meadows, shrublands and rocky ravines at altitudes of 3000–4900 m (Zheng, 2015). The species has long been recognized as endangered due to its narrow range, fragile habitat, and sensitivity to human disturbance, and has been listed as a globally vulnerable species by the International Union for Conservation of Nature (IUCN, 2020), an Appendix I species in the Convention on International Trade in Endangered Species of Wild Fauna and Flora (CITES, 2016), and a first class protected wildlife species in China. Moreover, recent studies have demonstrated Chinese Monal as an endemic bird species with the highest phylogenetic distinctiveness (Chen, 2013) and an umbrella species for alpine ecosystem conservation (Wang et al., 2017). Unfortunately, the species is still subjected to threats from livestock farming, poaching, and herb gathering, and its global population is estimated to be small and declining (BirdLife International, 2016). Since 2021, the Chinese government has started to protect the Chinese Monal as one of the 48 wildlife species that need to be rescued during the 14th Five-Year Plan period (National Forestry and Grassland Administration and National Development and Reform Commission, 2021). Therefore, there is an urgent need for further study and protective action to conserve this endangered species (Zhang et al., 2003; Xu et al., 2020).
The Chinese Monal is omnivorous but mainly forages plant foods (Lu et al., 1986; Zheng, 2015). Previous studies based on field observations have reported that this species mainly forages on young roots, stems, leaves, flowers, grass seeds, mosses and, occasionally, small invertebrates (He and Lu, 1985; Ma, 1988; Zhang, 1995). He et al. (1986) studied the diet of the Chinese Monal by analyzing the stomach contents of four specimens. However, this approach is not applicable in endangered wildlife researches under current legislation because it is fatal to the subjects, and has poor resolution in taxa identification (Forin-Wiart et al., 2018; McClenaghan et al., 2019). Furthermore, given that both the biotic and abiotic conditions of alpine ecosystems show pronounced seasonality, the food composition of Chinese Monal is expected to vary seasonally as well, yet no study has examined it. Thus, detailed information on the diet composition of the Chinese Monal and its seasonal variation has been quite scarce to date, largely constraining a thorough understanding and effective conservation of this endangered species.
In recent years, the DNA metabarcoding has been demonstrated as a useful tool for qualitative and quantitative analyses of animal diet composition due to its high-resolution and noninvasive sampling by using fecal samples (Pompanon et al., 2012; Shutt et al., 2021); therefore, it is especially ideal for endangered and elusive species when the collection of individuals is not practical (McClenaghan et al., 2019; Hacker et al., 2021). In this study, we investigated the plant diet composition of the Chinese Monal using a DNA metabarcoding approach on fecal samples that were collected from the central Qionglai Mountains, and compared the diet composition between the breeding season and non-breeding season to reveal the seasonal variations and facilitate conservation management plans.
The Qionglai Mountains are located in the north-central part of Sichuan Province, China. The mountains run in a general north-south direction and form the eastern edge of the Qinghai-Tibetan Plateau. This region supports a great diversity of natural ecosystems and wildlife species of conservation concerns, including Giant Panda (Ailuropoda melanoleuca), Golden Snub-nosed Monkey (Rhinopithecus roxellanae), Snow Leopard (Panthera unica), Takin (Budorcas taxicolor), and Dove Tree (Davidia involucrate). The alpine ecosystems throughout the Qionglai Mountains also form the core distribution range of the Chinese Monal (Xu et al., 2020).
The fecal samples were collected in five high-altitude mountain areas in the central Qionglai Mountains: Jiajin Mount (102°41ʹ E, 30°52ʹ N) and Borigou (102°45ʹ E, 30°54ʹ N) in Baoxing County, and Weijiagou (102°58ʹ E, 30°56ʹ N), Wenzhadu (103°0ʹ E, 30°55ʹ N), and Yangjiaowan (103°7ʹ E, 30°54ʹ N) in Wenchuan County (Fig. 1). These five areas, which are similar in topography, vegetation, and human disturbances, are supporting large Chinese Monal populations. We surveyed Chinese Monals throughout the alpine shrubs and meadows with altitudes ranging from 3300 m to 4200 m, and entered their habitat to search for feces once any individuals were detected. We only collected the part above the contact surface from relatively fresh feces to avoid the potential contaminations of soil, plants, or moss (McInnes et al., 2017). Each fecal sample was packaged into a single porous bag, and bags were collected in airtight boxes containing silica gel and stored at −20 ℃ prior to DNA extraction. To avoid to sample multiple feces from the same individuals, we adopted the following strategies: 1) when a single individual was detected, we collected only one fecal sample in its habitat; 2) when a group including multiple individuals was detected, the number of collected fecal samples was no more than the number of individuals in the group, and the fecal samples should be distinguishable with apparent differences in size, color, and/or shape; 3) for any detected individuals and groups, we sampled only once in one day. In total, we collected 215 fecal samples from Chinese Monals in the field from May 2019 to November 2020.
Total genomic DNA from the samples was extracted using the CTAB method (Zhang et al., 2006). DNA quality concentration and purity were monitored on 1% agarose gels. According to the concentration quantified using NanoDrop 2000c (Thermo Fisher Scientific, USA), DNA was diluted to 1 ng/μL using sterile water. We amplified a fragment of the second internal transcribed spacer (ITS2) of nuclear ribosomal DNA using the specific primers ITS3-2024F (forward: GCATCGATGAAGAACGCAGC) and ITS4-2409R (reverse: TCCTCCGCTTATTGATATGC) as the DNA barcode for identifying plants (Fahner et al., 2016; Moorhouse-Gann et al., 2018). All PCRs were carried out with 15 μL of Phusion High-Fidelity PCR Master Mix (New England Biolabs, USA), 0.2 μM of forward and reverse primers, and approximately 10 ng of template DNA. Thermal cycling consisted of initial denaturation at 98 ℃ for 1 min, followed by 30 cycles of denaturation at 98 ℃ for 10 s, annealing at 50 ℃ for 30 s, elongation at 72 ℃ for 30 s, and finally, 72 ℃ for 5 min. Mix same volume of 1 × loading buffer (contained SYB green) with PCR products and operate electrophoresis on 2% agarose gel for detection. PCR products were mixed in equidensity ratios. Then, mixture PCR products was purified with Qiagen Gel Extraction Kit (Qiagen, Germany). We collected total 215 fecal samples of Chinese Monals in the field, and finally, a total of 190 samples (88.4%) were successfully amplified.
The construction of libraries and high-throughput sequencing were performed at Novogene Incorporation (Beijing, China). In summary, the sequencing libraries were generated using the TruSeq DNA PCR-Free Sample Preparation Kit (Illumina, USA) (Chen et al., 2018; Jayawardena et al., 2018). DNA concentration was measured in a Qubit 2.0 Fluorometer (Thermo Fisher Scientific), and the library quality was assessed using the Agilent Bioanalyzer 2100 system. Finally, the libraries were sequenced on an Illumina NovaSeq 6000 platform and 250 bp paired-end reads were generated.
According to unique barcode sequence, paired-end reads was assigned to each sample, and the adaptor and barcode sequences were trimmed. Then, the resulting paired-end reads were merged using FLASH (V1.2.7, http://ccb.jhu.edu/software/FLASH/), and the final sequences were called tags. Once we generated high-quality tag sequences, we removed singletons and sequences below the minimum expected amplicon length (100 bp). All downstream analyses were based on the clean data described above. Compared to traditional Operational Taxonomic Unit (OTU) methods, ASV methods have been suggested to be better for metabarcoding data analyses, due to its higher taxonomic resolution and lower computational costs (Callahan et al., 2017). Therefore, we constructed the ASVs using usearch (v11.0) and the count table of ASVs were generated using vsearch 2.15.2 (—usearch_global —id 0.97) (Rognes et al., 2016). Then, we constructed a comprehensive ITS2 reference database. We searched NCBI GenBank (http://www.ncbi.nlm.nih.gov/nuccore) with the keywords 'ITS2 OR (internal transcribed spacer 2)' and extracted full-length ITS2 sequences with lengths ranging from 50 bp to 1 Mbp, which generated 525, 916 ITS2 sequences in total. Finally, we performed taxonomic assignment using BLASTn (version 2.7.1) by aligning the ASV sequences to the ITS2 reference database with a minimum identity of 97% and a minimum coverage of 80%. If one ASV was aligned to more than one taxon that had the same identity over the same coverage length, we reported the taxonomic category of the Lowest Common Ancestor (LCA) corresponding to these taxa.
In this study, we followed classification and nomenclature system of NCBI Taxonomy (https://www.ncbi.nlm.nih.gov/taxonomy), and considered all higher plants (including phylum Bryophytes, Pteridophytes, and Seed plants) in the analyses. Prior to analysis, we calculated the relative read abundance (RRA) for each assigned family/genus as the count of ASVs classified as the same family/genus divided by the total count of ASVs in a sample (including unclassified ASVs) (Kartzinel et al., 2015), and removed each rare family/genus with an RRA lower than 1% from each sample to minimize contaminants (Ando et al., 2018). Then, a final RRA matrix for the retained families/genera was calculated by excluding all rare families/genera and unclassified ASVs, and a corresponding matrix for the presence/absence of families/genera was generated. The matrices of family and genus were generated independently of each other.
The rarefaction curves with 84% confidence intervals (Gotelli and Colwell, 2011) for observed (interpolated) and extrapolated number of family/genus and Shannon's diversity against sample size were fitted based on the presence/absence matrices to compare diet diversity between breeding season (April–July) and non-breeding season (August–March) (Hsieh et al., 2016). Then, to determine the differences in diet composition between the breeding and non-breeding seasons, non-metric multidimensional scaling (NMDS) with Jaccard distances and Bray-Curtis distances were performed based on family/genus occurrence (binary presence/absence metrics) and relative read abundance (RRA matrices), respectively, and permutational multivariate analysis of variance (PERMANOVA) with 999 permutations (Anderson, 2001) were carried out as well. In addition, to illustrate the main diet composition during different periods, we calculated the frequency of occurrence of each family/genus by dividing the number of feces in which a family/genus occurred by the total number of feces for each period, and averaged the RRA of each family/genus across fecal samples for each period (Deagle et al., 2019; Chua et al., 2021; Chung et al., 2021). We identified the five families/genera with the highest frequencies of occurrence during the breeding and non-breeding seasons, respectively, and compared their RRAs using Mann-Whitney U tests. All statistical analyses were conducted in R 4.0.5 (R Core Team, 2021) using iNEXT (Hsieh et al., 2016), vegan (Oksanen et al., 2020), and ggplot2 (Wickham, 2009) packages. Unless otherwise stated, we reported means ± standard error, and an α-priori of p < 0.05 was considered significant.
In total, we successfully amplified 190 fecal samples of Chinese Monals, including 81 from the breeding season (April–July) and 109 from the non-breeding season (August–March). A total number of 19, 291, 480 read pairs were obtained from the 190 fecal samples, and 12, 636, 730 tags were retained after quality control. The average numbers of tags per sample were 69, 476.889 ± 1701.641 during the breeding season and 64, 303.688 ± 401.553 during the non-breeding season. The sequences were assigned to 8543 ASVs. The mean percentage of unclassified ASVs were low for each sample at both family (1.3% ± 0.3%) and genus levels (0.7% ± 0.2%). We identified 51 families and 131 genera of higher plants in total, but 16 families and 48 genera were completely absent from all samples after the removal of rare families and genera with RRAs lower than 1% from each sample (Appendix Table S1). Overall, the Chinese Monal fed 35 families and 83 genera of plants throughout the year.
In total, Chinese Monals fed 28 families and 62 genera of plants during the breeding season. At the family level, Brassicaceae was the highest in frequency of occurrence (96.3%), following by Apiaceae (42.0%), Poaceae (30.9%), and Ranunculaceae (30.9%; Appendix Table S2); while Cardamine accounted for the highest frequency of occurrence (95.1%), following by Acanthocalyx (27.2%) and Angelica (25.9%) at the genus level (Appendix Table S3). Herbaceous plants dominated the diet, accounting for 72.6% of the total genera, while only 1 tree-obligate genus (1.6%) and 5 shrub-obligate genera (8.1%) were consumed by the species (Appendix Table S3).
During non-breeding season, Chinese Monals consumed 31 families and 66 genera of plants. Family Brassicaceae had the highest frequency of occurrence (91.7%), followed by Poaceae (45.9%) and Apiaceae (39.5%; Appendix Table S2); and genus Cardamine (87.2%), Poa (45.0%), Megacarpaea (33.9%), and Meconopsis (33.9%) were the highest in frequency of occurrence (Appendix Table S3). Among the consumed genera, over 70.0% were herbaceous plants, and only 3 tree-obligate genera (4.5%) and 6 shrub-obligate genera (9.1%) were fed (Appendix Table S3).
Both the mean number of the family and genus detected in each sample from the breeding season (3.827 ± 0.250 families and 4.593 ± 0.324 genera) were slightly lower than those from the non-breeding season (4.459 ± 0.215 families and 5.413 ± 0.262 genera). However, the rarefaction curves suggested that the overall number (Fig. 2A, C) and Shannon's diversity (Fig. 2B, D) of the family and genus, regardless of actual or double (extrapolated) sample sizes, did not vary significantly between breeding and non-breeding seasons, indicating by an overlap of their 84% confidence intervals (Gotelli and Colwell, 2011). Multivariate analyses using NMDS illustrated that diet composition distributions of Chinese Monals during the breeding and non-breeding seasons were partially partitioned for both occurrence and RRA at the family and genus levels (Fig. 3). Furthermore, PERMANOVA revealed significant differences between periods for both the occurrence (family: R2 = 0.041, P = 0.001; genus: R2 = 0.063, P = 0.001) and RRA (family: R2 = 0.024, P = 0.002; genus: R2 = 0.034, P = 0.001) of the diet composition.
The top five plant families and genera with the highest frequency of occurrence varied between periods. Brassicaceae was the most prevalent family in both breeding and non-breeding diets; Apiaceae and Poaceae had the second-highest frequency of occurrence in breeding and non-breeding seasons, respectively; Caprifoliaceae was common in breeding season only whereas Papaveraceae was common in non-breeding season only (Fig. 4A). At the genus level, Cardamine was the most prevalent diet in both periods; whereas Acanthocalyx was common in the breeding season but scarce in non-breeding season; conversely, Meconopsis, Draba, and Megacarpaea had a higher frequency of occurrence only in the non-breeding season (Fig. 4B). Mann-Whitney U tests showed that at the family level, the RRAs of Brassicaceae and Caprifoliaceae were significantly higher in the breeding season than in the non-breeding season, whereas those of Poaceae and Papaveraceae were significantly lower (Fig. 4C; all P < 0.05); the RRAs of genera Cardamine, Acanthocalyx, and Anemone were significantly higher in the breeding season than in the non-breeding season, whereas those of Poa, Meconopsis, Draba, and Megacarpaea were significantly lower (Fig. 4D; all P < 0.05).
Our study represents the first attempt to demonstrate the plant diet of the Chinese Monal based on DNA metabarcoding of feces. We identified the diet composition at a fine resolution and determined that the yearly diet of the Chinese Monal was composed of at least 35 families and 83 genera of higher plants. In comparison to previous field observations, this finding extensively expands knowledge on the Chinese Monal's diet (He et al., 1986; Lu et al., 1986; Ma, 1988; Zhang, 1995), suggesting that it has a much wider breadth in diet than previously thought. Compared to studies using traditional microhistological analysis of the plant diets of some confamilial species, such as Tibetan Snowcock (Tetraogallus tibetanus) (Jia et al., 2021), Hazel Grouse (Bonasa bonasia) (Xu et al., 2015), and Blood Pheasant (Ithaginis cruentus) (Luo et al., 2016), and the congener Sclater's Monal (Lophophorus sclateri) (Luo et al., 2012b, 2016), we detected a much more diverse diet composition for the Chinese Monal. Furthermore, the other studies failed to distinguish Bryophyta plants despite their high proportions (Jia et al., 2021; Luo et al., 2016), further indicating the noticeable advantages of DNA metabarcoding methods for studying animal diets (Shao et al., 2021).
We found that the Chinese Monal predominantly forages on herbaceous plants, which is highly adaptive to its foraging habitat and behavior, as it prefers to forage in alpine meadows and shrublands above tree lines (He et al., 1986), and it has a weak flight ability and typically forages on the ground. Meanwhile, 10 genera of Bryophytes were detected in Chinese Monals' fecal samples, and some of them had high frequencies of occurrence especially during the breeding season (Appendix Table S3), indicating that mosses are an important part of this species' diet. Generally, mosses are not food items for most herbivores due to their low digestibility; nevertheless, consuming mosses has been reported in several Galliformes species (Martin and Hik, 1992; Luo et al., 2012a, b, 2016; Jia et al., 2021) and other vertebrate taxa (Prins, 1982). A possible explanation for this is that mosses are rich in arachidonic acid not found in other phyla of higher plants, which might improve the cold resistance of animals surviving in cold environments (Prins, 1982; Guo et al., 2020).
Despite similar diversity, significant seasonal variations in diet composition of the Chinese Monal were found, suggesting high foraging plasticity of this species. The cyclic changes in biomass and availability of different food-source plant driven by particular plant phenology in the alpine ecosystems are likely responsible for these observed variations. As the most prevalent plant throughout the Chinese Monal habitat, the genus Cardamine from the family Brassicaceae thrives in moist areas during spring and summer, providing abundant and accessible food resources for the species during the breeding season. Most of the herbaceous plants start to wither in autumn, and thick snow covers a large area of habitat in winter, which largely reduces food availability and accessibility during the non-breeding season. The Chinese Monal may shift its foraging strategy to overcome food deficiencies, by increasing the consumption of herbs that are relatively more accessible. For instance, the genus Poa from family Poaceae have long stems and seeds that can last longer and are not easily covered by snow, thus become primary food resources with high frequency of occurrence and RRA for the species during non-breeding season. In addition, it feeds more subterranean parts (roots and subterranean stems) of herbaceous plants by digging and pecking foods in the soil using its beak and feet (Ma, 1988), which likey increased the importance of plants with thick roots (such as the genus Meconopsis from family Papaveraceae and the genus Megacarpaea from family Brassicaceae) in the diet of Chinese Monal during this period.
Based on the diet composition revealed in our study, there are two findings with Chinese Monal conservation implications. It has been reported that the species forages on many bulbs of the Fritillaria spp. (family Liliaceae) in summer, and some stomach content studies have confirmed this finding (He et al., 1986; Long et al., 1998). However, we did not find any ASVs belonging to the genus Fritillaria in our study. Previous studies demonstrated that DNA metabarcoding based on the ITS2 sequence is effective in identifying Fritillaria spp. (Luo et al., 2012a; Gao et al., 2018; Zheng et al., 2019). And our additional analyses further provided reliable evidence that our primer set do work for genus Fritillaria, as we successfully aligned our primer sequences to the Fritillaria ITS sequences in our reference database. Meanwhile, we aligned the raw sequencing tags to the ITS2 reference database using BLASTn and no alignments to Fritillaria ITS2 sequences were found, suggesting that the missing of genus Fritillaria in our fecal samples is not due to the removal of potential Fritillaria ITS2 sequences in the pre-process filtering steps. Therefore, we speculate that the absence of Fritillaria species in our samples is most likely resulting from the intensive gathering of Fritillaria spp. in our study area, rather than the false negative results of the research methods. The bulbs of Fritillaria spp. are valuable materials in traditional Chinese medicine, and local people extensively and repeatedly collect Fritillaria bulbs in our study areas every year, resulting in a substantial reduction in this important food resource for the species (Wang et al., 2017). Thus, the gathering of Fritillaria spp. might represent a local threat to Chinese Monal populations, and we suggest further studies to determine the nutritional function of Fritillaria spp. on Chinese Monal and the effect of Fritillaria gathering on the food resources of the species, and restricting this activity in Chinese Monal habitats to facilitate species conservation.
Free-ranging yaks throughout the Qinghai-Tibet Plateau have long been recognized as a severe threat to Chinese Monal conservation because they were expected to contribute to habitat loss for the Chinese Monal (He et al., 1986; Yu et al., 2017). Our study implies that there is potential food competition between the two species, as some of the main diet components of the Chinese Monal, such as plants from the Brassicaceae, Poaceae, and Ranunculaceae families, are also preferred by the yaks (Yao et al., 2011; Teng et al., 2013, 2014). Intensive yak grazing in alpine meadows might lead to a notable reduction in food abundance for the Chinese Monal; thus, for further protection of Chinese Monal populations and habitats, rigorous control of grazing intensity and area is suggested.
Animal fecal samples collected in the field may have various sources of contaminants, which could lead to errors and biases in molecular dietary analyses (McInnes et al., 2017). In particular, contamination errors are riskier in plant diet analysis, as non-food plant tissues are quite easily mixed into fecal samples from soil, water, or nearby plants. In this study, we reduced potential contaminants by removing rare genus ASVs with RRAs lower than 1% from each sample (Ando et al., 2018). This resulted in the exclusion of 16 families and 48 rare genera; among them, 8 genera of Bryophytes were likely contaminants from wet ground surfaces rather than foraged by the Chinese Monal initiatively; some genera were apparently artificially induced contaminants that were not plants naturally found in the Chinese Monal habitat, such as Gossypium (Malvaceae), Citrus (Rutaceae), Cannabis (Cannabaceae), Nicotiana and Capsicum (Solanaceae); and the others might represent items that were seldomly foraged by the species (Appendix Table S1). This procedure substantially reduced ASVs that are likely to be contaminants, non-food items, or at least, non-preferred food items, accounting for a negligible proportion of the Chinese Monal's diet. Therefore, we advocate for procedures that remove rare ASVs or OTUs in wildlife diet studies using metabarcoding methods, which are useful to control the errors induced by unavoidable contaminants and allow us to concentrate our focus on important ecological information (i.e., primary diet composition).
BW and CZ conceived and designed the experiments, YL and JY conducted the field sampling, BW, YL and GZ performed the experiments and drafted the earlier version of the paper, CD, HH, LZ and XX contributed in data analysis and paper review. All authors read and approved the final manuscript.
The present study complies with the current laws of China. Fecal sample collection is non-invasive and has not caused any damages to any animals.
The authors declare that they have no competing interests.
We are grateful to Jundong He, Shanshan Xia, Weijia Ye, Tianxiang Zhou and Hualin Yang for their assistance in the field work. This study was supported by Department of Wildlife Conservation, National Forestry and Grassland Administration of China, the National Natural Science Foundation of China (32000354), the Special Foundation for National Science and Technology Basic Research Program of China (2018FY100701), and the Sichuan Science and Technology Program.
Supplementary data to this article can be found online at https://doi.org/10.1016/j.avrs.2022.100034.
Anderson, M.J., 2001. A new method for non-parametric multivariate analysis of variance. Austral Ecol. 26, 32-46.
|
Gotelli, N.J., Colwell, R.K., 2011. Estimating species richness. In: Magurran, A.E., McGill, B.J. (Eds.), Frontiers in Measuring Biodiversity. Oxford University Press, New York, pp. 39-54.
|
Guo, L., Liu, F.Q., Shi, L., Wen, L.Y., 2020. Physiological and ecological adaptability of Blood Pheasant (Ithaginis cruentus) and its favorite Bryophyte. Chin. J. Appl. Environ. Biol. 26, 1400-1405. (in Chinese).
|
He, F.Q., Lu, T.C., 1985. Ecology of the Chinese Monal in winter. Zool. Res. 6, 345-352. (in Chinese).
|
He, F.Q., Lu, T.C., Lu, C.L., Cui, X.Z., 1986. Study on the breeding ecology of the Chinese Monal. Acta Ecol. Sin. 6, 186-192. (in Chinese).
|
Long, T.L., Shao, K., Guo, G., Cheng, C.Y., Zou, X.Y., Landel, H., et al., 1998. Field tracking and ecological observation of the Chinese Monal in winter. Sichuan J. Zool. 17, 104-105. (in Chinese).
|
Lu, T.C., Liu, R.S., He, F.Q., Lu, C.L., 1986. Ecological studies on Chinese Monal. Acta Zool. Sin. 32, 273-279. (in Chinese).
|
Luo, K., Ma, P., Yao, H., Song, J., Chen, K., Liu, Y., 2012a. Molecular identification of Fritillariae cirrhosae Bulbus and its adulterants. World Sci. Technol. Modern. Tradit. Chin. Med. Materia Medica 14, 1153-1158. (in Chinese).
|
Luo, X., Wu, T.P., Huang, A.Q., 2016. Diet analysis and foraging strategy of two sympatric pheasants at Mt. Gaoligong in winter. Chin. J. Ecol. 35, 1003-1008. (in Chinese).
|
Luo, X., Xu, M.Y., Wu, L.X., 2012b. Spring diets and food nutrients of Sclater's Monal at Mt. Gaoligong, China. Sichuan J. Zool. 31, 17-22. (in Chinese).
|
Ma, G.Y., 1988. Observation on the Chinese Monal in Gansu Province. Sichuan J. Zool. 7, 41-42. (in Chinese).
|
McClenaghan, B., Nol, E., Kerr, K.C.R., 2019. DNA metabarcoding reveals the broad and flexible diet of a declining aerial insectivore. Auk 136, 1-11.
|
Teng, L.W., Li, Y.X., Liu, Z.S., Dong, J.P., Yao, Z.C., Zhang, J.T., 2013. Summer diet composition of feral yak in Helan Mountains. J. Econ. Anim. 17, 192-196. (in Chinese).
|
Teng, L.W., Wang, Y., Li, L.Y., Yao, Z.C., Lu, P.F., Li, Y.X., et al., 2014. Autumn diet composition of feral yak (Bos grunnieus) in Helan Mountains. J. Anhui Agr. Sci. 42, 6258-6260. (in Chinese).
|
Wickham, H., 2009. ggplot2: elegant graphics for data analysis. Springer-Verlag, New York.
|
Xu, L.X., Wu, W., Ma, S.G., Ma, J., Cheng, K., 2015. Winter food habits of Hazal Grouse (Bonasa bonasia) in Liangshui National Nature Reserve. Chinese J. Wildl. 36, 402-406. (in Chinese).
|
Yao, Z.C., Liu, Z.S., Wang, Z.D., Hu, T.H., Li, Z.G., 2011. Winter and spring diet composition of feral yak in Helan Mountains, China. Acta Ecol. Sin. 31, 673-679. (in Chinese).
|
Yu, X., Chen, J.C., Wang, B., Yan, Y., Ran, J.H., He, F., et al., 2017. Population density estimation and habitat suitability assessment of Lophophorus lhuysii during breeding season in Xiaozhaizigou National Nature Reserve, Sichuan Province. Sichuan J. Zool. 36, 361-367. (in Chinese).
|
Zhang, B.W., Li, M., Ma, L.C., Wei, F.W., 2006. A widely applicable protocol for DNA isolation from fecal samples. Biochem. Genet. 44, 503-512.
|
Zhang, T., 1995. Distribution and ecology of Chinese Monals in Baishuijiang Nature Reserve, Gansu. Chinese J. Zool. 30, 25-28. (in Chinese).
|
Zheng, G.M., 2015. Pheasants in China. Higher Education Press, Beijing.
|
Zheng, H., Deng, K.Y., Chen, A.Q., Fu, S.B., Zhou, D., Wang, W.W., et al., 2019. Molecular identification and genetic relationship of Fritillaria cirrhosa and related species based on DNA barcode. Acta Pharm. Sin. 54, 2326-2334. (in Chinese).
|