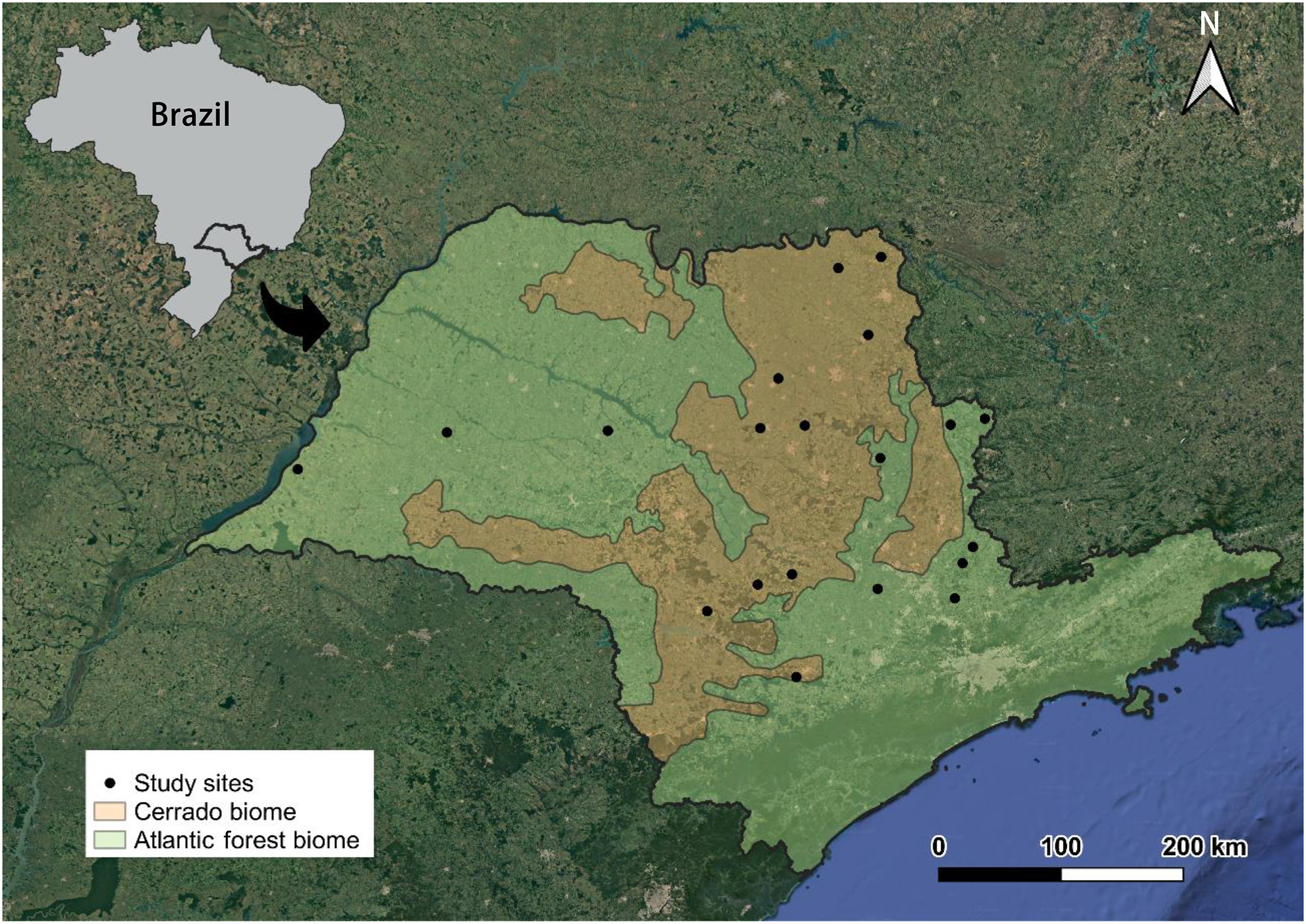
Citation: | Ana B. Navarro, Juliano A. Bogoni, Marcelo Z. Moreira, Luís F. Silveira. 2023: Intraguild niche partitioning in granivorous birds from the late past. Avian Research, 14(1): 100075. DOI: 10.1016/j.avrs.2023.100075 |
Niche partitioning is a widespread ecological strategy within trophic guilds, ensuring the coexistence of sympatric species by reducing interspecific competition. Stable isotope analysis of carbon and nitrogen and isotopic niche metrics (width and overlap) are used as a proxy to investigate niche partitioning among species of a guild. In our study, we investigated if niche partitioning was an ecological mechanism contributing to the coexistence of granivorous birds from the same geographic region along time, employing an isotopic approach. We sampled and isotopically analyzed (δ13C and δ15N values) wing feathers from 58 specimens of granivorous birds collected between 1900 and 1966 in southeastern Brazil. We grouped birds according to the main habitat type used by them (forest: Cyanoloxia brissonii, Leptotila rufaxilla, and Leptotila verreauxi; and grassland: Columbina squammata and Sicalis flaveola). We used the Isotopic Richness (IRic) metric to measure the isotopic niche width and the Isotopic Similarity (ISim) and Isotopic Nestedness (INes) metrics to measure the niche overlap between groups and species. The results of low isotopic niche overlap suggest that common granivorous birds had a clear niche partitioning in southeastern Brazil in the late past, especially through foraging in distinct habitat types (forest and grassland; ISim = 0.1, INes = 0.2). Niche overlaps in almost all species-by-species comparisons were low (ISim ≤0.3, INes ≤0.4), except between C. brissonii and L. verreauxi (ISim = 0.6, INes = 1.0). These results suggest that these birds, belonging to the same trophic guild, coexisted through niche and resource partitioning. Despite being considered a plastic trophic guild, the isotopic niche partitioning among granivorous birds indicates that each of these species uses a set of specific resources and habitats. This raises an ecological concern about the homogenization of landscapes across the Neotropics, oversimplifying food resources and habitats to granivorous birds.
Sympatric species may compete for food resources, habitat, nutrients, and any other factor essential to their existence (Schoener, 1983). Community ecology theories suggest that niche partitioning is an ecological and evolutionary strategy widely distributed within biological communities, reducing the effects of interspecific competition and enabling species to coexist (Hutchinson, 1959). Niche dimensions potentially present adjustments, being displaced over evolutionary history and habitat changes, prompting direct consequences on the structuration of biological communities from short to long term (Hutchinson, 1957). Competing species may partition their niches through differential use of (micro) habitat, resources, or time (MacArthur, 1958; Tilman, 1982; Cotton, 1998). Assessing the occurrence of this strategy in community ecology may not be trivial, but a potential solution is to use metrics that measure the niche width and niche overlap between species (Chase and Leibold, 2003). The concept of niche width refers essentially to the diversity of resource use shown by an organism, in which the restricted and specialized use of resources produces narrow niches and, conversely, generalist organisms that exploit a wider range of resources have broad niches (Roughgarden, 1972). The niche overlap concept denotes the degree of resource sharing between organisms in the same habitat and, possibly, a competition for such resources (Pielou, 1972).
The use of carbon and nitrogen stable isotope analysis in ecological studies specifically related to species niche is a well-established field in the scientific literature (e.g., Bubadué et al., 2021; Gaspar et al., 2022; Magioli et al., 2022; Xu et al., 2022). Measuring the ratio of stable isotopes of carbon (12C and 13C; δ13C) in an animal's tissue provides robust information about its habitat use, while the ratio of stable isotopes of nitrogen (14N and 15N; δ15N) is a good predictor of the animal diet (Kelly, 2000; Navarro et al., 2022). Through the bivariate union of δ13C and δ15N values, it is possible to access two important axes fundamental to the species niche (diet and habitat use), in which the δ-space has similarities to the n-dimensional space of the classical niche theory proposed by G. E. Hutchinson (Newsome et al., 2007). After the association between the classical and the isotopic niche theories, some isotopic metrics were proposed to gather information about the width and overlap of the species niche (Jackson et al., 2011; Cucherousset and Villéger, 2015; Eckrich et al., 2020). These metrics, associated with knowledge about the natural history of the species, may help to infer the ecological strategies and processes present in communities, such as niche partitioning and coexistence (Botta et al., 2018; Manlick and Pauli, 2020; Silverthorne et al., 2020; Bubadué et al., 2021).
Trophic guilds are groups of species that have similar diets, which may result in interspecific competition for shared resources (Root, 1967). The coexistence among species of a guild in the same geographic region is possible under some conditions: ⅰ) if the abundance and availability of resources are sufficient to provide the species' requirements; ⅱ) if intraspecific competition is stronger than the interspecific competition; or ⅲ) if there is niche partitioning (habitat, resources, or time) among the species (Schoener, 1974; Rathcke, 1976; Connell, 1983; Powers and McKee, 1994).
The Neotropics harbor a rich diversity of birds occurring in sympatry and belonging to many trophic guilds (Kissling et al., 2012). Among the widely distributed and species-rich guilds, neotropical granivorous birds can occupy distinct habitat types (e.g., forests, savannas, shrublands, grasslands, and even anthropogenic areas, such as urban and arable lands) and have a diet specialized in the consumption of seeds (IUCN, 2022). It is common for species of this guild to be neglected in conservation efforts, as they are highly associated with the also neglected non-forest ecosystems in Brazil (Lopes et al., 2010; Overbeck et al., 2015). In agricultural areas, granivores are eventually considered a pest, once some of them may eat the seeds of cultivated plants, hindering crop productivity (Branco et al., 2016). Nonetheless, granivorous birds have important roles in the maintenance of ecological processes, such as seed predation (Pizo and Vieira, 2004). In the Neotropics, this guild have been negatively impacted by human expansion into natural areas over the last century (Navarro et al., 2021). In the landscapes of southeastern Brazil, anthropogenic impacts have occurred for centuries, but human activities have intensified after the 1970s, with increased use of fertilizers and pesticides (Franco and Pelaez, 2016; FAO, 2019; IFA, 2019), low percentage of forest cover remaining (Kronka et al., 2005; Victor et al., 2005), and homogenization of landscapes by agriculture (MapBiomas, 2022). Understanding the historical ecology of species is relevant to gaining knowledge about their natural history before this intensification of anthropogenic impacts, clarifying the ecological patterns and processes of communities under the low human disturbance conditions of the past.
In this study, we investigated if niche partitioning was an important ecological mechanism for the coexistence of historical individuals (i.e., collected until the 1960s) of regionally widespread granivorous birds from southeastern Brazil. Using stable isotopes of carbon (δ13C) and nitrogen (δ15N), and calculating metrics of the isotopic niche (width and overlap), we assessed whether species from the granivorous guild employed the niche partitioning strategy to coexist by reducing their competition for food resources and habitat along time. In doing so, we linked operational definitions of niche partitioning using stable isotopes for a plastic trophic guild, pointing to theoretical implications of stable coexisting during a historic period that precedes the rapid acceleration of the environmental degradation of South American biomes.
We sampled 58 specimens of granivorous birds that were historically collected (1900–1966) and deposited at the Museum of Zoology, University of São Paulo, Brazil (Appendix A). These historical individuals were collected in a period that precedes an intensification of anthropogenic impacts on the landscapes of southeastern Brazil. After the 1970s, there were at least three glaring socio-environmental phenomena: (1) the substantial increase in fertilizers and pesticides uses across — thenceforth — dominant agricultural landscape in the region (Franco and Pelaez, 2016; FAO, 2019; IFA, 2019); (2) the stabilization of deforestation rates in the state of São Paulo, given that a low parcel of forest cover remained (Kronka et al., 2005; Victor et al., 2005); and (3) the high homogenization of landscapes composed mainly of sugarcane crops and pastures, complemented by the overwhelming expansion of urban areas (MapBiomas, 2022).
Individuals were originally collected across 20 municipalities in the state of São Paulo, Brazil (Fig. 1; Appendix Fig. S1). Ten of these localities are located in the Atlantic Forest biome and the remaining are in the Cerrado biome. The Atlantic Forest is composed of different vegetational classes, with the Atlantic seasonal semideciduous forest predominant across the ten study sites. The Cerrado is also composed of distinct classes of vegetation, being strongly similar to the structure of the Atlantic seasonal semideciduous forest in the state of São Paulo (Durigan et al., 2007). We tested whether the biome was related to the δ13C and δ15N values of the birds before proceeding with the statistical analysis (see section 2.4). We also tested whether the isotopic values of birds varied over the years, considering the large timescale of the samples (see section 2.4).
Among the variety of families that congregate granivorous birds, we studied species of Cardinalidae, Columbidae, and Thraupidae, families that comprise a good representation of the native species richness of this guild in the Neotropics. We measured the carbon and nitrogen isotopic values of five species of this guild: Cyanoloxia brissonii (Lichtenstein, 1823), Columbina squammata (Lesson, 1831), Leptotila rufaxilla (Richard and Bernard, 1792), Leptotila verreauxi (Bonaparte, 1855), and Sicalis flaveola (Linnaeus, 1766). The Ultramarine Grosbeak (C. brissonii) is a species widely distributed in South America between the Amazon basin and the Patagonian steppe, inhabits the interior and edge of forests, shrubs and disturbed habitats, and is a sought-after species for the illegal trade (Brewer, 2020). The White-tipped Dove (L. verreauxi) is the widest-ranging native Columbid, occurring from southern Texas to a wide distribution in South America, and it is common in disturbed woodlands, forest edges and shrubby clearings (Giese et al., 2020). The Gray-fronted Dove (L. rufaxilla), although not as widely distributed as its congener, can be found throughout mainland South America, preferring the interior of forests, particularly humid ones, but sometimes is encountered on forest edge and in clearings (Baptista et al., 2020a). The Scaled Dove (C. squammata) has its largest population distributed from northeastern Argentina to northeastern Brazil, and it is a resident of arid savanna and scrub, but also found on second growth and riparian thickets (Baptista et al., 2020b). The Saffron Finch (S. flaveola) has its largest population distributed from northeastern Brazil to central Argentina, and although it is commonly kept as caged-birds, it is very adaptable to human-modified habitats and also found in a wide variety of natural environments (Rising and Jaramillo, 2020). Besides the similarities in diet and geographic distribution of these granivores, the habitat use is quite similar among some species, especially when referring to forest edges — highlighting the importance of assessing niche partitioning among these birds.
Besides species-by-species analyses, we also grouped birds according to the main habitat types used by them (forest and grassland). Based on observations of the species' natural history, we defined forest granivores (C. brissonii, L. rufaxilla, and L. verreauxi) as those species that are more restricted to the interior and the edge of forests, while the grassland birds (C. squammata and S. flaveola) are those species found in open areas and shrublands. For habitat grouping, we also used the mean carbon isotopic values of species, given that individuals foraging in grasslands have higher δ13C values compared to forest individuals.
We sampled the second primary flight feather from the individuals' left wing to run the isotopic analysis. The feather is a metabolically inert tissue, providing information about the habitat use and dietary patterns of the individual during the molting period, which usually takes weeks to months (Bearhop et al., 2003). In Neotropical species, it is common that the molting cycle occurs annually during the rainy season and after the reproductive period (Piratelli et al., 2000; Marini and Durães, 2001). A small cut (approximately 0.5 cm2) in the feather's vane, not including the rachis, is sufficient to obtain the isotopic values of carbon (δ13C) and nitrogen (δ15N), without causing visible damage to the museum specimens (Wiley et al., 2017). We cleaned the feathers with 70% alcohol to eliminate surface contaminants and did not employ any lipid treatment since feather is a low-lipid tissue. The samples were packed in tin capsules (5 × 3.5 mm) and sent for isotopic analysis at the Laboratory of Isotopic Ecology at the Center for Nuclear Energy in Agriculture, University of São Paulo, Brazil.
We then analyzed the samples with a Continuous Flow Isotope Ratio Mass Spectrometry, using a Carlo Erba CNH-1110 elemental analyzer coupled to a Thermo Scientific Delta Plus mass spectrometer, converting and purifying the sample into gases. This process transforms the carbon of the sample into CO2 and the nitrogen into N2; hence, the mass spectrometer provides isotopic values expressed in delta (δ) per mill (‰) notation, following the equation: δ13C or δ15N = [(Rsample/Rstandard) – 1], multiplied by a thousand, and where R is the corresponding ratio of 13C/12C or 15N/14N of the sample gases. We calibrated the machine using reference materials (NBS-19 and NBS-22 for carbon and IAEA-N1 and IAEA-N2 for nitrogen) to reference our samples with international scales (V-PDB for carbon and atmospheric N2 for nitrogen). We employed a replication procedure for 10% of the samples and the analytical precision showed a standard deviation of 0.09‰ for both isotopes.
After the isotopic analysis, we corrected the individual values of δ13C by decade, considering the Suess effect. This process describes the decline in the δ13C values of the atmosphere due to the input of anthropogenic CO2 on Earth, mainly as a result of the increased burning of fossil fuels (Revelle and Suess, 1957). We used data from the literature to obtain values of δ13C atm per decade from 1900 to 1960, the timescale of our samples (Francey et al., 1999). We also corrected the values of δ13C and δ15N according to the isotopic discrimination of each species, which is the difference between consumer tissue and food (Hobson and Clark, 1992). To do so, we calculated the trophic discrimination factors (TDFs) of carbon and nitrogen for each species by using the SIDER package in R, which estimates TDFs based on a phylogenetic regression model (Healy et al., 2018).
To ensure that individuals collected in different biomes could be grouped regardless of their locality, we compared the mean values of δ13C and δ15N between birds from the Cerrado and the Atlantic Forest using the non-parametric Wilcoxon test. Comparison of mean δ13C and δ15N values between biomes was employed by grouping individuals by habitat (forest and grassland birds) and also by species. Additionally, we used the mean annual rainfall rates as a proxy for environmental variation among the localities where our samples were collected. We tested the correlation between the mean annual rainfall and isotopic values (δ13C and δ15N, separately) for all individuals together and by habitat-grouping, using the Spearman's correlation test. We also ensured that individuals collected in different years could be grouped using Spearman's correlation test; whereby we first calculated the correlation between the isotopic values (δ13C and δ15N, separately) of all granivorous birds and the year of collection. Subsequently, we grouped the individuals by habitat (forest and grassland species) and tested the correlation between the year of collection and the isotopic values. We also tested the correlation between year and isotopic values by species, assessing whether there is any taxonomic effect.
Before calculating the isotopic metrics, we standardized the multidimensional space by scaling the carbon and nitrogen isotopic values of the individuals in a range from 0 to 1. Thus, we ensured that the isotopic axis with the largest range did not contribute disproportionally — in mathematical terms — for the calculation of the niche metrics. The metric Isotopic Richness (IRic) measures the total convex hull area (i.e., niche width) filled by individuals of a group or species (Cucherousset and Villéger, 2015). The Isotopic Similarity (ISim) and Isotopic Nestedness (INes) metrics are complementary and both are used to calculate the overlap of isotopic space between groups or species, where values close to 0 indicate low niche overlap and values close to 1 show high overlap (Cucherousset and Villéger, 2015). We calculated the IRic metric for groups by habitat (forest and grassland granivorous birds) and for the five species (C. brissonii, C. squammata, L. rufaxilla, L. verreauxi and S. flaveola). We also calculated the overlap metrics (ISim and INes) between groups and among all the species. Before calculating the metrics, we employed a bootstrap procedure (1000 runs) to randomize the individuals of the species with the largest sample size, parameterizing according to the species with the smallest sample size. Thus, we corrected any sample size bias that might influence the isotopic metrics.
To complement and corroborate the niche width and overlap analyzes described above, we also employed the Bayesian methodology of the SIBER package (Jackson et al., 2011). The Bayesian standard ellipse analysis uses 40% of the data regardless of sample size to calculate the core isotopic niche area. We compared the proportionalities of niche width values of the Bayesian Standard Ellipse Area (SEAb) and the metric IRic. In the Bayesian methodology there is also a niche overlap analysis based on posterior estimates, providing the percentage of overlap between two ellipses, which we used to compare with the ISim values. The iterations of the Bayesian model to calculate the SEA for each group generate 40,000 posterior values, which were used to run a t-test to compare whether the niche width between forest and grassland granivores were significantly different.
We calculated the means and standard deviations of δ13C and δ15N values for each species and group by habitat. Due to data (a)symmetry, we used both parametric and non-parametric tests to compare the δ13C and δ15N values. In doing so, we employed a Wilcoxon test to investigate the isotopic differences between forest and grassland granivorous birds. We also compared the isotopic values among forest species (ANOVA with Tukey post hoc test for δ13C; and Kruskal-Wallis test with Wilcoxon post hoc for δ15N), and between the grassland species (t-test for both δ13C and δ15N). We used the script provided by Cucherousset and Villéger (2015), which employs the 'geometry', 'ape' and 'rcdd' packages in R software version 4.1.2, to calculate the isotopic metrics. All the statistical analyses were also run in the same version of R (R Core Team, 2022).
The mean of δ13C and δ15N of individuals collected in different biomes (Cerrado and Atlantic Forest), either grouped by habitat (forest and grassland; Appendix Fig. S2) or by species (Appendix Fig. S3), were not statistically different (Appendix Table S1). We also found no significant correlation between mean annual rainfall rates and isotopic values by locality for any of the tested groups (all individuals: δ13C – ρ = −0.11, p = 0.41; δ15N – ρ = −0.13, p = 0.34; only forest species: δ13C – ρ = −0.03, p = 0.86; δ15N – ρ = −0.19, p = 0.32; and only grassland species: δ13C – ρ = −0.08, p = 0.66; δ15N – ρ = 0.08, p = 0.68). The correlation test between the year of sample collection and isotopic values (δ13C and δ15N, separately) did not present a significant result for any of the tested correlations (all individuals grouped, only forest species, only grassland species and by species; Appendix Table S2). All these results ensured that grouping individuals collected in distinct localities and years was robust and did not bias the consecutive results.
The comparison of the Isotopic Richness (IRic) between groups by habitat showed that grassland granivores had a larger niche width than forest granivores (Fig. 2), and the overlap of isotopic niches (ISim and INes) between these groups was low (ranging from 0.1 to 0.2; Table 1). The comparison of the mean values of SEAb between forest (SEAb = 16.4‰2) and grassland granivores (SEAb = 19.5‰2) showed a significant difference in their niche widths (t (7839) = −39.2, p < 0.01) and no Bayesian niche overlap was found between them (Appendix Fig. S4). The mean values of δ13C and δ15N between forest and grassland granivores were significantly different for both comparisons (δ13C: W = 40, p < 0.01; δ15N: W = 155, p < 0.01; Table 2).
Comparison | ISim | INes | |
Species by habitat | Forest birds vs Grassland birds | 0.1 | 0.2 |
Forest birds | C. brissonii vs L. rufaxilla | 0.1 | 0.2 |
C. brissonii vs L. verreauxi | 0.6 | 1.0 | |
L. rufaxilla vs L. verreauxi | 0.1 | 0.4 | |
Grassland birds | C. squammata vs S. flaveola | 0.3 | 0.4 |
Forest vs Grassland birds | C. brissonii vs C. squammata | 0.0 | 0.1 |
L. rufaxilla vs C. squammata | 0.0 | 0.0 | |
L. verreauxi vs C. squammata | 0.1 | 0.2 | |
C. brissonii vs S. flaveola | 0.0 | 0.0 | |
L. rufaxilla vs S. flaveola | 0.0 | 0.0 | |
L. verreauxi vs S. flaveola | 0.0 | 0.1 |
n | Mean δ13C (‰) | SD δ13C | Mean δ15N (‰) | SD δ15N | ||
Species by habitat | Forest birds | 28 | −22.0 | 3.3 | 3.5 | 1.5 |
Grassland birds | 30 | −13.7 | 3.4 | 5.5 | 2.0 | |
Forest birds | C. brissonii | 9 | −21.4 | 2.7 | 3.7 | 1.4 |
L. rufaxilla | 9 | −24.0 | 1.9 | 2.9 | 1.2 | |
L. verreauxi | 10 | −20.8 | 4.2 | 3.7 | 1.9 | |
Grassland birds | C. squammata | 15 | −14.6 | 3.7 | 5.0 | 1.9 |
S. flaveola | 15 | −12.9 | 3.0 | 6.0 | 2.0 |
The metrics calculated for each of the species individually showed that the niche width (IRic) was quite similar among granivores, except for L. rufaxilla which had its niche ca. 2.5 times smaller than its congener L. verreauxi (Fig. 3). The results of the ISim and INes metrics in the species-by-species comparisons showed low overlap in isotopic niches among most granivores, except in the comparison between the forest birds C. brissonii and L. verreauxi that showed almost complete niche overlap (Table 1). The niches measured by the SEAb values showed a similar proportionality in the widths (Appendix Fig. S4), in which the species of the genus Leptotila presented the largest and smallest niche widths (SEAbL. rufaxilla = 7.4‰2 and SEAbL. verreauxi = 27.7‰2) and the other granivores showed intermediate values (SEAbC. brissonii = 13.5‰2, SEAbC. squammata = 17.6‰2 and SEAbS. flaveola = 18.4‰2). The proportions of Bayesian overlap between species were also low (C. brissonii vs. L. rufaxilla = 10%; L. rufaxilla vs. L. verreauxi = 10%; C. squammata vs. S. flaveola = 20%; all other comparisons = 0%), except between C. brissonii and L. verreauxi, which showed an intermediate overlap of 40%. The mean values of δ13C and δ15N among the forest species were not significantly distinct (δ13C: H = 4.64, p = 0.1; δ15N: F = 0.74, p = 0.49; Appendix Fig. S5). The same is true for grassland species, which showed no differences between their means (δ13C: t = −1.36, p = 0.19; δ15N: t = −1.37, p = 0.18; Appendix Fig. S5).
Niche partitioning is an important ecological mechanism that permits the coexistence of species with similar habits by reducing their spatiotemporal competition for resources (MacArthur, 1958). Species from the same trophic guild, which eat the same type of food, often partition their niches so that they coexist in the same region (Balciauskas et al., 2021). Using isotopic metrics as a proxy to measure ecological niche width and overlap, we identified that common granivorous birds — collected by naturalists during the first half of the 20th century — had a clear niche partitioning in southeastern Brazil, especially through foraging in different habitat types (forest or grassland). Considering that the isotopic niche — composed of the values of δ13C and δ15N — captures in its axes information related to diet and habitat use by an organism (Newsome et al., 2007), metrics that measure the isotopic niche overlaps between groups or species may provide information about shared use of resources — pinpointing to possible interspecific competition (Bosenbecker and Bugoni, 2020; Silverthorne et al., 2020).
The low niche overlap, measured by Isotopic Similarity (ISim) and Isotopic Nestedness (INes), showed that forest and grassland granivorous birds hardly competed for resources, enabling their coexistence. We also showed that the historical niche of grassland granivores was larger than the niche of forest granivores, certainly related to a greater diversity of seeds available in native open areas. A high species richness of herb-shrub plants, many providing seeds for birds, is associated with savannas and shrublands in the Neotropics (Sano et al., 2008; Amaral et al., 2017), benefiting species that forage in this habitat. Yet, there is a severe decline in the biodiversity across these phytogeographic regions (e.g., Cerrado and/or interior plateaus of semidecidous Atlantic Forest), given that over 40% of the Cerrado biome has been converted into agriculture in the last decades (Klink and Machado, 2005; Colli et al., 2020), changing dramatically the isotopic niche of neotropical birds (Navarro et al., 2021).
Our results reveal that the isotopic niche width of each granivore species composed of historical specimens did not differ greatly, except for L. rufaxilla that had the smallest niche. Niche overlaps in almost all species-by-species comparisons were low, except between C. brissonii and L. verreauxi. Niche partitioning is usually observed when reducing dietary overlap in the presence of a potential competitor (Schluter, 1982). Thus, our results suggest that these potential competitor bird species, belonging to the same trophic guild, coexisted in the same localities through niche and resource partitioning, denoting the stable coexisting during a historic period that precedes the overwhelming degradation of South American biomes. The Leptotila ground-doves showed well-marked differentiations in their isotopic spaces. For instance, the niche of L. rufaxilla was approximately 2.5 times smaller than that of the tolerant to disturbed habitats, widespread and common L. verreauxi, showing low niche overlap between them. Congeneric species may have similar foraging strategies and habitat use, but show a low similarity in niche and use of food resources, ensuring the coexistence of these closely related birds in the same regions (Guaraldo et al., 2016, 2019). This seems to be similar to forest doves of the genus Leptotila, which occurred in symtopy in our study, but evolutionarily differentiated their niches to explore distinct food resources and microhabitats. L. rufaxilla is more restricted to the forest interior (Ingels, 1982), where it captures food items, as observed by the mean value of δ13C. Nevertheless, L. verreauxi is frequently observed in the forest edges and anthropogenic areas (Ingels, 1982), with some individuals plastically able to eat in open areas, as denoted by the higher mean value of δ13C for this species. Additionally, L. rufaxilla is often considered in the literature as a specialist in diet and habitat use compared to other granivores (Morante-Filho et al., 2015; dos Anjos et al., 2019), even though it is fairly common in the forests of southeastern Brazil (e.g., Andrade and Marini, 2002; Cavarzere et al., 2010; Alexandrino et al., 2013). The specialization of L. rufaxilla may be confirmed by the smaller niche width compared to the other species.
The only exception to the clear niche partitioning among historical granivore species was the comparison between C. brissonii and L. verreauxi, which showed an almost complete overlap between their isotopic niches. This result suggests a shared use of the same food resources and habitat, which could increase competition between these granivores. Nonetheless, niche overlaps do not always indicate strong interspecific competition, if there is sufficient abundance and availability of the shared resources in the environment (Morin, 2011). Classic ecological theory predicts that when the intraspecific competition is stronger than interspecific competition a pair of species are able to coexist (Connell, 1983) — despite literature reviews finding little support for this pattern (Adler et al., 2018). Moreover, interspecific competition is frequently highly asymmetric, with different consequences for both species (Connell, 1983). Under a strong intraspecific competition for shared resources in short supply there may be consequences for individuals (e.g., decline in per capita body size or fitness) but allowing the persistence with closely related species (Morris, 1959), often being promoted by species rarity. Thus, even if the food resources and habitat used are highly similar, two species may not have a niche overlap by adapting-behavior to explore different time periods, microhabitats and/or foraging strata (MacArthur, 1958; Pianka, 1974). Despite that, we acknowledge that this information could not be accessed by using isotopic data, highlighting the importance of basic natural history in supporting our knowledge of species foraging habits. L. verreauxi is a forest dove that forages predominantly on the ground, while C. brissonii is a passerine that captures its food both in the understory and on the forest ground (Wilman et al., 2014). Thus, this could be evidence that these species were not strong competitors and stably coexisted in communities in southeastern Brazil, despite the high isotopic niche overlap that we found. Additionally, generalist species usually have wide niches due to the plasticity in dietary requirements of the individuals within a population, increasing niche overlap with species of similar foraging habits (Dammhahn et al., 2017). L. verreauxi, a generalist forest species, had the largest niche width among all birds and C. brissonii also had a wide niche, which may have determined a greater niche overlap between these species without representing a high competition for resources, but suggesting generalist individuals within the community.
Even though L. verreauxi and C. brissonii could show resource and niche partitioning in the past (e.g., distinct strata used to forage), the result of the high overlap between these birds raises an alert for their current coexistence if there is a reduction in the availability and abundance of shared resources. Common forest granivorous birds have had their isotopic niches reduced due to the advance of anthropogenic impacts, especially land-use changes, in southeastern Brazil (Navarro et al., 2021). There are several factors derived from the human expansion that directly drive populations to decline or even to be extinct locally (e.g., fragmentation, habitat loss, urbanization; Gibson et al., 2013; Chase et al., 2020; Schneiberg et al., 2020). Additionally, species within a community may offer negative and selective filters that can lead to the disappearance of competitors (Schoener, 1983). There is an ongoing population decline of C. brissonii, mainly driven by the illegal trade of this species (Destro et al., 2012). If this passerine increases its competition with L. verreauxi for resources and habitat, already depleted in the study sites due to anthropogenic impacts, this may represent an additional selective filter for the local survival of the species.
These historical ecological results are relevant as a landmark for future studies with birds facing constant anthropogenic pressure. It is likely that grassland granivores have increasingly similar diets following the current homogenization of landscapes, while forest granivores have their niches increasingly reduced, due to the depletion of habitat and resources. We will only be able to test these predictions if we build a solid groundwork of knowledge about past ecology, using museum specimens and feathers collected over time, and then monitor future ecological changes in the species. Thus, our study further emphasizes the importance of the ongoing collection of voucher specimens through the years, providing data to long-timescale ecological and evolutionary studies. The use of museum specimens in studies of isotopic ornithology is a potential to be explored, especially in regions with a known history of human expansion, such as the Neotropics.
Historically, common granivorous bird species used the niche partitioning strategy, reducing potential competition for food resources and habitat. Using δ13C and δ15N values as a proxy to calculate the isotopic niche, we observed that forest and grassland granivorous birds hardly competed for resources, enabling the coexistence of species from these groups. Even within groups that forage in the same habitat, there is a low overlap of isotopic niches among species (except between C. brissonii and L. verreauxi). This result suggests a clear niche partitioning among granivores, indicating a different use of food resources in the past century across southeastern Brazil. Over a millennial-scale, these granivorous birds have adapted their behavior to equalize the intraspecific competition and minimize the interspecific competition, but since the Columbian era in the last five centuries, human-induced influences have caused profound rearrangements on Neotropical landscapes inducing cascade-effects upon biota ranging from local extinctions to environmental eutrophication, causing ecological consequences on species coexistence and biodiversity. Despite being considered a plastic trophic guild and tolerant to many anthropogenic impacts, the isotopic niche partitioning among species indicates that each one of them uses a unique set of specific resources and habitat. This raises an ecological concern about the homogenization of landscapes — consequently to the oversimplification of food resources and habitat to granivorous birds — across the Neotropical realm.
Conceptualization: A.B.N.; Formal analysis: A.B.N.; Investigation: A.B.N. and J.A.B.; Methodology: A.B.N. and M.Z.M.; Writing – original draft: A.B.N. and J.A.B.; Writing – review & editing: all authors; Resources: L.F.S. and M.Z.M; Supervision: L.F.S. and M.Z.M. All authors read and approved the final manuscript.
The authors declare that they have no known competing financial interests or personal relationships that could have appeared to influence the work reported in this paper.
We are immensely grateful to all bird collectors and ornithologists who dedicated their lives to building a historical database of bird specimens, found deposited in the Bird Collection of the Museu de Zoologia da Universidade de São Paulo, Brazil. ABN thanks the São Paulo Research Foundation (FAPESP) for the doctoral scholarship (process 2020/07619-0) and LFS thanks the Conselho Nacional de Pesquisa e Desenvolvimento Científico e Tecnológico (CNPq) for the research grant (no. 302291/2016-6 and 308337/2019-0). JAB is supported by the São Paulo Research Foundation (FAPESP) postdoctoral fellowship grants 2018-05970-1 and 2019-11901-5.
All samples were collected under Brazilian laws (SISBIO 10013-2, process no. 27899-1; CGen 13123/2015, Resolution 10/2018 and 23/2019) and approved by Ethics Committee (227/2015).
Supplementary data to this article can be found online at https://doi.org/10.1016/j.avrs.2023.100075.
Alexandrino, E.R., Bovo, A.A.A., da Luz, D.T.A., da Costa, J.C., Betini, G.S., de Barros Ferraz, K.M.P.M., et al., 2013. Aves do campus "Luiz de Queiroz" (Piracicaba, SP) da Universidade de São Paulo: mais de 10 anos de observações neste ambiente antrópico. Atual. Ornitol. 173, 40–52.
|
Botta, S., Secchi, E.R., Rogers, T.L., Prado, J.H.F., de Lima, R.C., Carlini, P., et al., 2018. Isotopic niche overlap and partition among three Antarctic seals from the western Antarctic Peninsula. Deep-Sea Res. Pt. Ⅱ 149, 240–249.
|
Branco, J.O., Keske, B.R., Barbieri, E., 2016. Abundance and potential impact of granivorous birds on irrigated rice cultivation, Itajaí, Santa Catarina, Brazil. Arq. Inst. Biol. 83, e0462014.
|
Chase, J.M., Leibold, M.A., 2003. Ecological Niches: Linking Classical and Contemporary Approaches. The University of Chicago Press, Chicago.
|
Cotton, P.A., 1998. Temporal partitioning of a floral resource by territorial hummingbirds. Ibis 140, 647–653.
|
Destro, G.F.G., Pimentel, T.L., Sabaini, R.M., Borges, R.C., Barreto, R., 2012. Efforts to combat wild animals trafficking in Brazil. In: Akeem, L.G. (Ed.), Biodiversity Enrichment in a Diverse World. IntechOpen, London, pp. 421–436.
|
Ingels, J., 1982. Separation in the field of white-fronted and grey-fronted doves (Leptotila verreauxi and Leptotila rufaxilla). Bonner Zool. Beiträge 33, 61–70.
|
Klink, C.A., Machado, R.B., 2005. A conservação do Cerrado brasileiro. Megadiversidade 1, 147–155.
|
Kronka, F.J.N., Nalon, M.A., Matsukuma, C.K., Kanashiro, M.M., Ywane, M.S.S. -I., Barradas, A.M.F., et al., 2005. Monitoramento da vegetação natural e do reflorestamento no Estado de São Paulo. Anais XII Simpósio Brasileiro de Sensoriamento Remoto 16–21, 1569–1576.
|
Morin, P.J., 2011. Community Ecology, second ed. Wiley-Blackwell, Chichester.
|
Piratelli, A.J., Siqueira, M.A.C., Marcondes-Machado, L.O., 2000. Reprodução e muda de penas em aves de sub-bosque na região leste de Mato Grosso do Sul. Rev. Bras. Ornitol. 8, 99–107.
|
R Core Team, 2022. R: a Language and Environment for Statistical Computing. R Foundation for Statistical Computing, Vienna.
|
Revelle, R., Suess, H.E., 1957. Carbon dioxide exchange between atmosphere and ocean and the question of an increase of atmospheric CO2 during the past decades. Tellus 9, 18–27.
|
Sano, S.M., Almeida, S.P., Ribeiro, J.F., 2008. Cerrado: Ecologia e Flora. Embrapa Informação Tecnológica, Brasília.
|
Silverthorne, K.M., Perrig, P.L., Lambertucci, S.A., Pauli, J.N., 2020. Isotopic niche partitioning in avian scavenger communities of South America. J. Raptor Res. 54, 440–446.
|
Tilman, D., 1982. Resource Competition and Community Structure. Princeton University Press, Princeton.
|
Victor, M.A.M., Cavalli, A.C., Guillaumon, J.R., Serra Filho, R., 2005. Cem Anos de Devastação: Revisitada 30 Anos Depois. Ministério do Meio Ambiente, Brasília.
|
Wiley, A.E., James, H.F., Ostrom, P.H., 2017. Emerging techniques for isotope studies of avian ecology. In: Webster, M.S. (Ed.), The Extended Specimen: Emerging Frontiers in Collections-Based Ornithological Research. CRC Press, Boca Raton, pp. 89–109.
|
Wilman, H., Belmaker, J., Simpson, J., de la Rosa, C., Rivadeneira, M.M., Jetz, W., 2014. EltonTraits 1.0: species-level foraging attributes of the world's birds and mammals. Ecology 95, 2027–2027.
|
Xu, X., Jiang, J., Lei, Y., Wang, C., Qing, B., Ding, C., 2022. Using stable isotope to compare the habitat use and trophic level between the new and old breeding range of wild Crested Ibis in the early breeding season. Avian Res. 13, 100007.
|
Comparison | ISim | INes | |
Species by habitat | Forest birds vs Grassland birds | 0.1 | 0.2 |
Forest birds | C. brissonii vs L. rufaxilla | 0.1 | 0.2 |
C. brissonii vs L. verreauxi | 0.6 | 1.0 | |
L. rufaxilla vs L. verreauxi | 0.1 | 0.4 | |
Grassland birds | C. squammata vs S. flaveola | 0.3 | 0.4 |
Forest vs Grassland birds | C. brissonii vs C. squammata | 0.0 | 0.1 |
L. rufaxilla vs C. squammata | 0.0 | 0.0 | |
L. verreauxi vs C. squammata | 0.1 | 0.2 | |
C. brissonii vs S. flaveola | 0.0 | 0.0 | |
L. rufaxilla vs S. flaveola | 0.0 | 0.0 | |
L. verreauxi vs S. flaveola | 0.0 | 0.1 |
n | Mean δ13C (‰) | SD δ13C | Mean δ15N (‰) | SD δ15N | ||
Species by habitat | Forest birds | 28 | −22.0 | 3.3 | 3.5 | 1.5 |
Grassland birds | 30 | −13.7 | 3.4 | 5.5 | 2.0 | |
Forest birds | C. brissonii | 9 | −21.4 | 2.7 | 3.7 | 1.4 |
L. rufaxilla | 9 | −24.0 | 1.9 | 2.9 | 1.2 | |
L. verreauxi | 10 | −20.8 | 4.2 | 3.7 | 1.9 | |
Grassland birds | C. squammata | 15 | −14.6 | 3.7 | 5.0 | 1.9 |
S. flaveola | 15 | −12.9 | 3.0 | 6.0 | 2.0 |